4 January 2021 by ShahriarLahouti. Last update 10 February 2023.
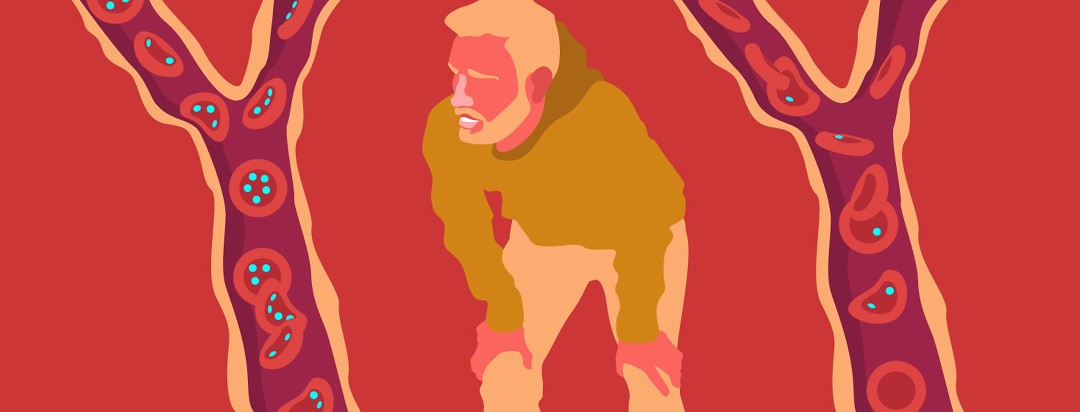
CONTENTS
- Preface
- Definition and diagnostic criteria
- Anatomic backbone
- Respiratory control
- Physiologic backbone
- Mechanism of tissue hypoxia
- Respiratory distress syndrome
- Differential diagnosis
- Approach to diagnosis and management
- Appendix
- Going further
- References
Preface
Respiratory distress is a clinical term and refers to the patient’s subjective sensation of dyspnea with signs indicating difficulty breathing (i.e. increased work of breathing). Patients with respiratory Failure are in extremes with severe respiratory distress and profound derangement in arterial oxygenation or CO2 elimination. This represents a life-threatening group of disorders for which inadequate management may lead to rapid clinical deterioration. Before a deep dive into management of these critical patients, the groundwork should be laid.
In the following discussion the basic physiology of gas exchange and signs and symptoms of respiratory distress are explored.
Definition and diagnostic criteria
Background
- In the following figure a general view on O2 and CO2 transport cascaded is illustrated (figure 1)1 2.
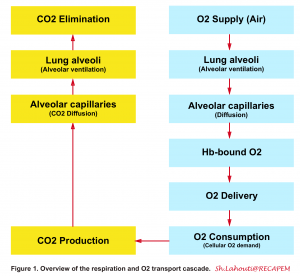
Definition
- From this perspective, acute respiratory failure is defined by the sudden onset of severe impairment of gas exchange (type I, hypoxemic respiratory failure or type II hypercapnic respiratory failure) or insufficient pulmonary function to keep up with increased ventilatory demands associated with systemic hypermetabolism such as sepsis (type IV respiratory failure) 3.
- In more practical terms, acute respiratory failure is recognized when two or more clinical features of respiratory distress are combined with one or more laboratory features listed below (figure 2).
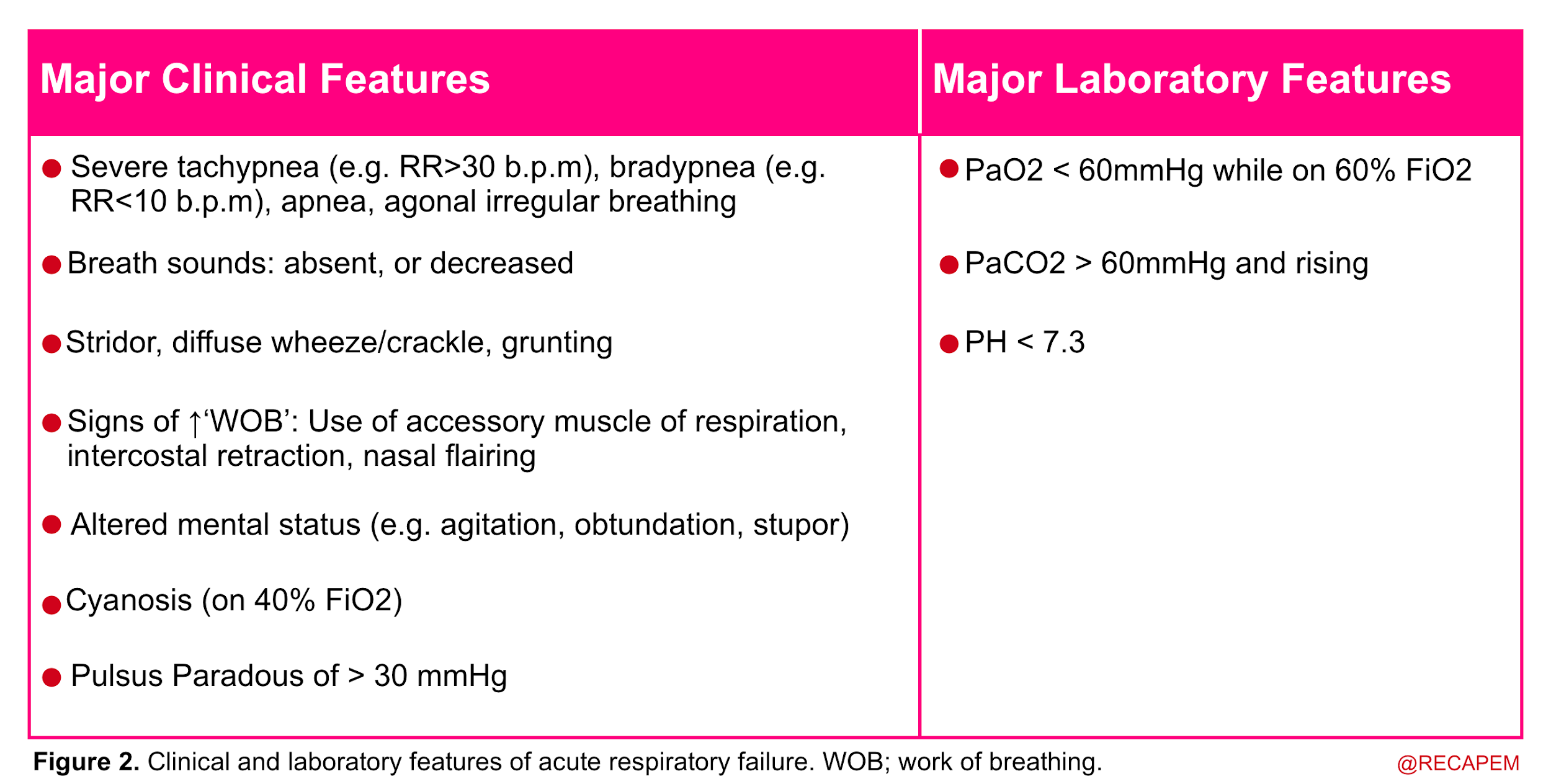
Anatomic backbone
Respiratory apparatus
- The respiration (ventilation) is governed by the integrated function of respiratory apparatus which is composed of the following compartments (figure 3):
- Controller
- Central neurons (in medulla) which control rate, depth and pattern of breathing upon information which is received from central and peripheral receptors.
- Peripheral nerves (wiring)
- The neural impulses from the central nervous system traverse the spinal cord and motor neurons, reaching and activating the diaphragm and other respiratory muscles.
- Thoracic cage and respiratory muscles (bellows)
- Contraction of the respiratory muscles creates negative pleural pressure by expanding the chest cavity and pushing the abdominal contents down. The negative pressure created in the thorax during inspiration leads to subatmospheric pressure in the alveoli, creating a gradient for the flow of inspired air toward the alveoli. During expiration the chest wall contracts and a positive pressure is built up within the airways which blow off the air.
- Respiratory tract
- The respiratory system is divided into two parts based on their structure and function. The first is the conducting part (from nose to terminal bronchiole); an organized array of progressively smaller cartilaginous airways through which the bulk of the air is moved into and out of the lung during ventilation. The second is the respiratory part; a non-cartilaginous collection of thin-membrane walls (figure 4).
- Alveolar capillary unit
- It consists of the respiratory bronchioles, the alveolar ducts, the alveoli, and the pulmonary capillary bed. Gas exchange occurs in the alveoli through a dense meshlike network of capillaries and alveoli called the alveolar–capillary network (figure 5).
- Controller
From this perspective dyspnea results from a mismatch between central respiratory control activity and incoming afferent information from receptors in the airways, lungs, and chest wall structures (figure 3)4. The sensation of dyspnea is often due to a combination of these factors.
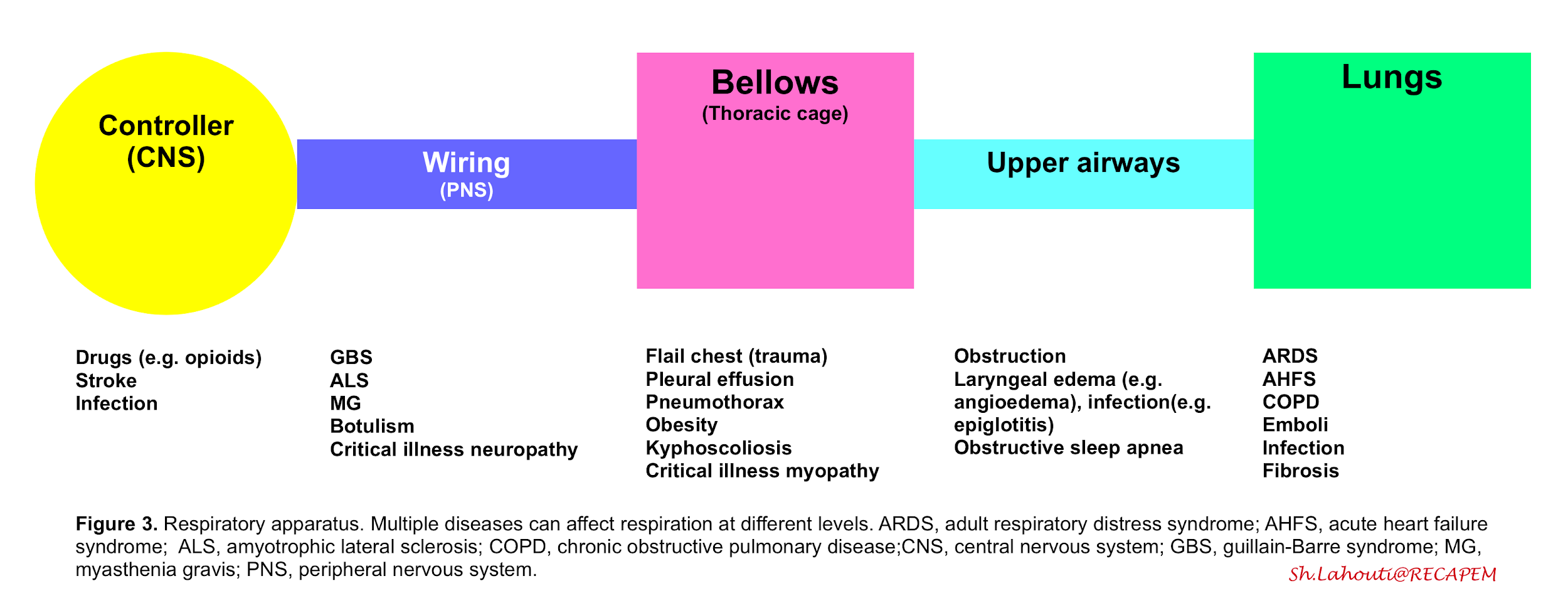
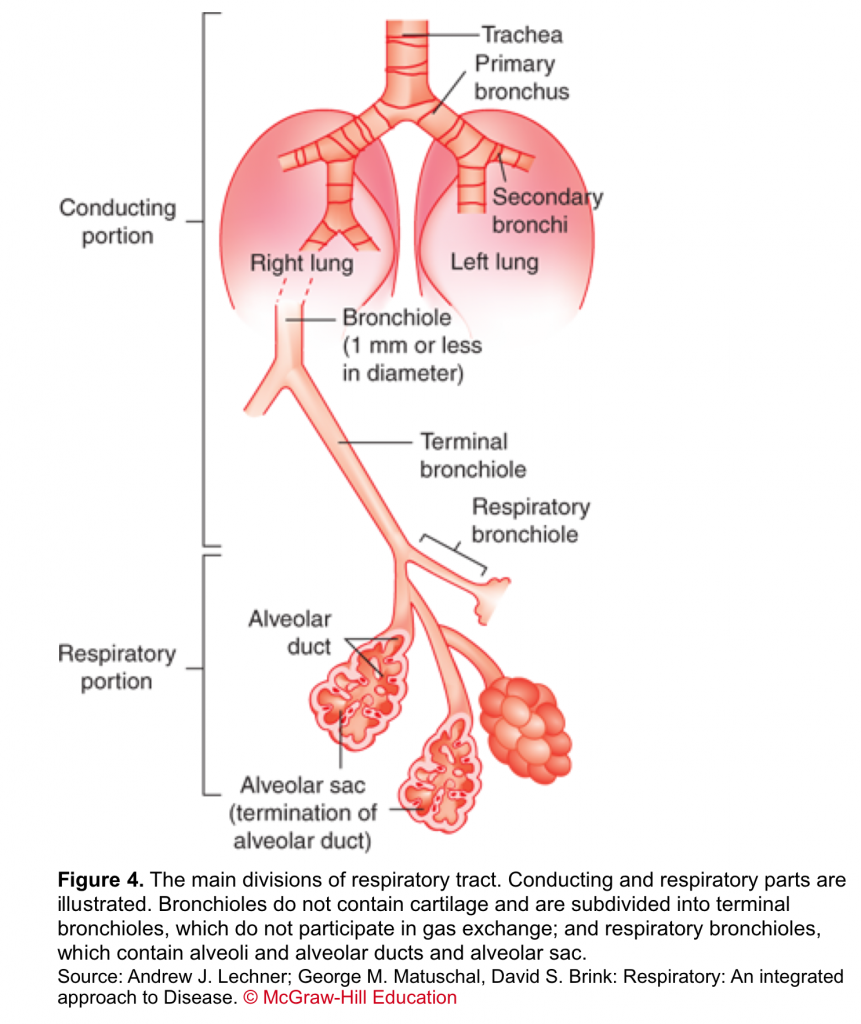
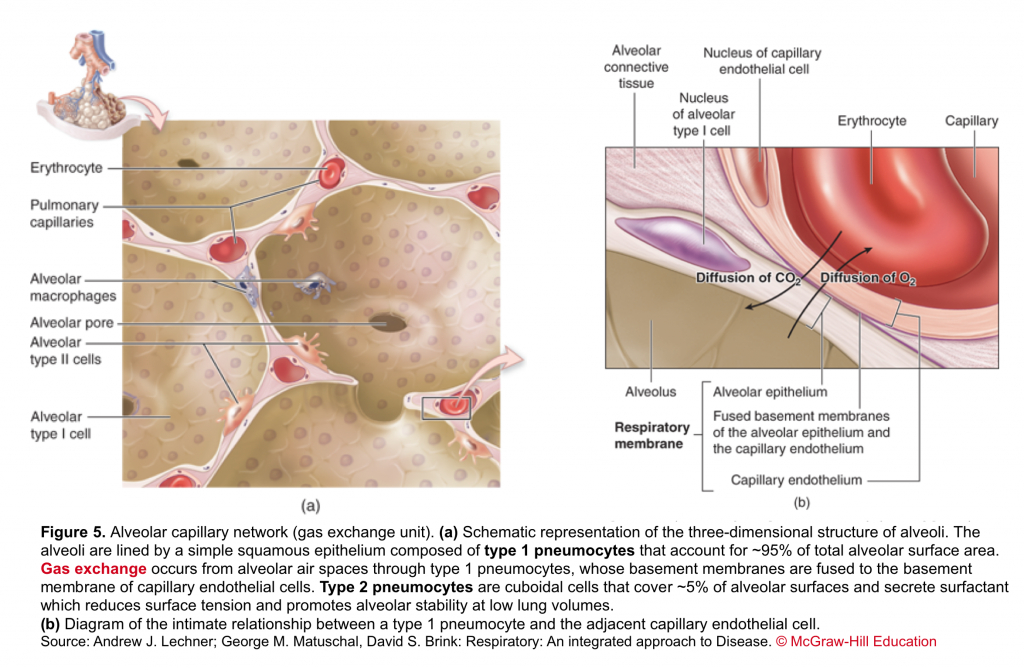
Respiratory control
- Refers to the generation and regulation of rhythmic breathing by the respiratory center in the brainstem and its modification by the input of information from higher brain centers and systemic receptors (figure 6)5.
- Chemoreceptors which primarily send signal regarding PCO2 and PO2 tension and are located both centrally (medulla) and peripherally (carotid and aortic bodies)
- Mechanoreceptors are distributed within the airways, pulmonary parenchyma and chest wall. These are activated by different stimuli and their afferent signals to the central nervous system will affect the breathing pattern in different ways.
By enlarge, the predominant stimulus for driving the respiratory center is hypercapnia (figure 7) which mainly operates via central chemoreceptors. Hypoxemia is generally a weak stimulus to drive the respiratory center.
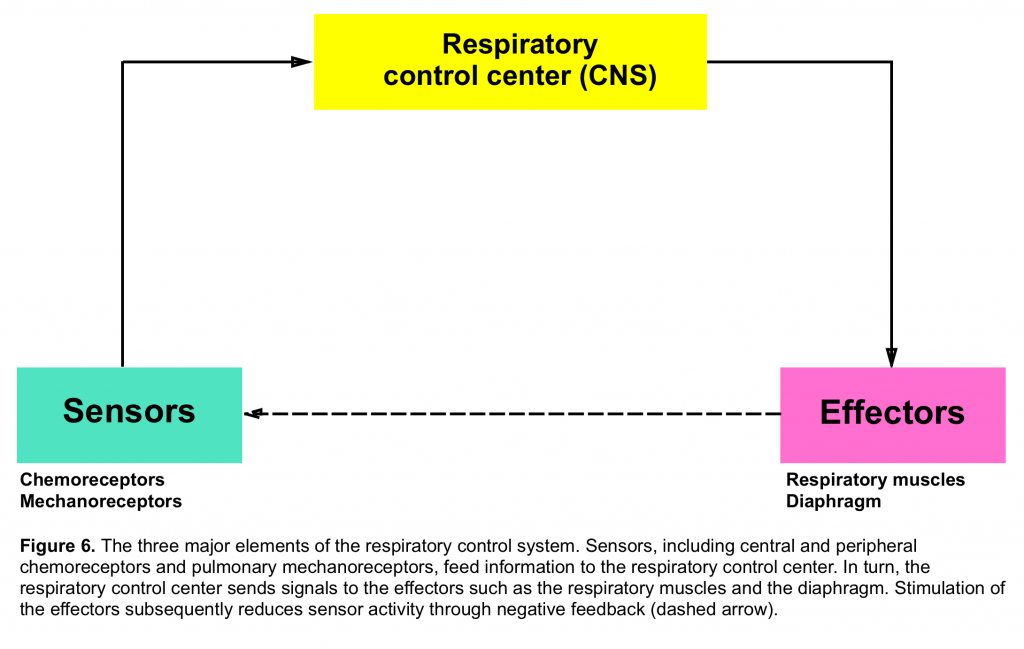
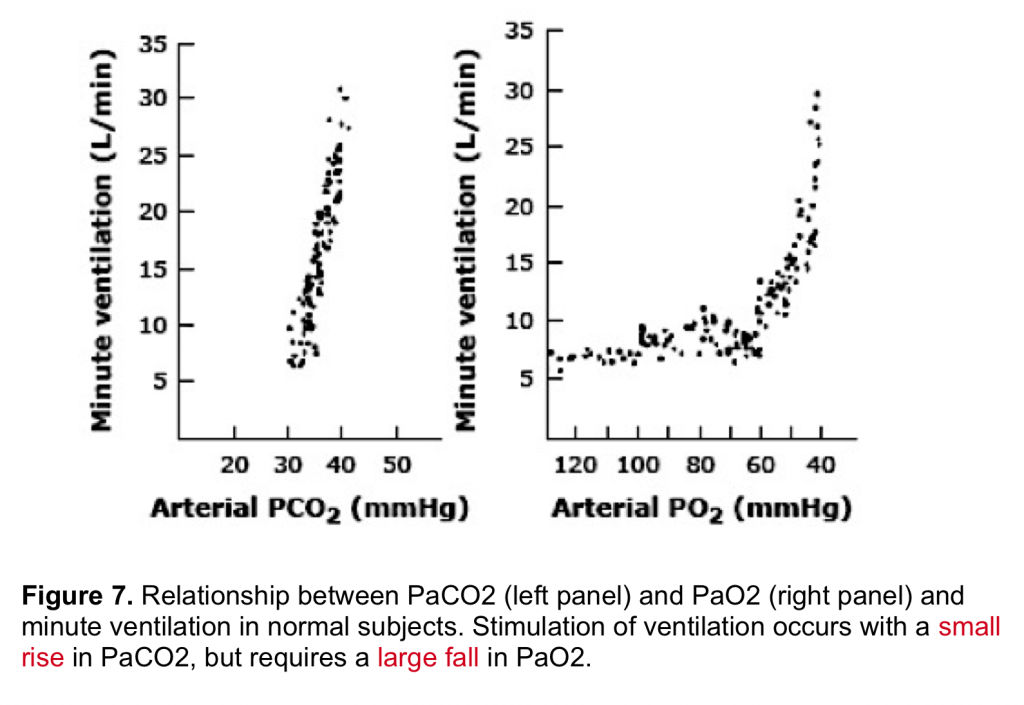
- Respiration is for the most part an active process. Work of breathing (WOB) is the energy expended to inhale and exhale the breathing air. Respiratory muscles should overcome two opposing force against respiration:
- Elastic force (volume-change related force): The work (mainly during inspiration) that is required to overcome elastic recoil of the lung and chest wall.
- Dynamic force (flow-related; i.e. resistance to air flow):Generally there is a frictional resistance to gas flow and a pressure difference is required to overcome this resistance. The work that it takes for respiratory muscle to provide this pressure difference and maintain air flow is therefore another component of the breathing work.
- Factors that can lead to increased work of breathing
- Increased metabolic demand: Hypercapnia (the predominant stimuli) and hypoxemia drives the central respiratory controller and consequently either the rate or depth of breathing is altered.
- Non-compliant Lung and chest wall: A healthy alveolus is like a beachball 🎈that is easy to inflate and deflate; while a non-compliant unit is like a basketball 🏀 which takes more effort to get inflated and deflated. In conditions where lungs are stiffened (e.g. alveolar filling disorders such as pulmonary edema, interstitial pulmonary fibrosis, hyperinflation seen in obstructive lung disease etc) or chest wall is noncompliant as in massive obesity,pleural effusion; it takes tremendous effort for respiratory muscle to inflate/deflate the lungs and therefore work of breathing is increased.
- Increased resistance to the airflow: Remember the relationship of flow to diameter of airway (flow ~ r4 ). When the diameter of the airway is ↓, the flow Is ↓ to the power Of four. In order to provide an appropriate flow in a narrowed airway, it takes tremendous work of breathing to generate flow.
Physiologic backbone
Ventilation
Ventilation is the bulk movement of gases in and out of the lungs via the conducting part of the respiratory tract. Ventilation is the algebraic product of respiratory rate, multiplied by the amount of air moved per breath, termed the tidal volume (VT). Keep in mind that only a portion of each VT enters the alveoli (alveolar ventilation, VA) where diffusion can occur, while the remaining portion of VT is confined to anatomical regions unsuitable for diffusion, collectively called the dead space volume (VD)6. With each tidal breath (≈500cc), there’s ≈100-150 cc of air which do not meet the gas-exchange unit (anatomic dead space). Normally, dead space ventilation represents 20% to 30% of the minute ventilation5.
Relevant terms and definitions
- 👉Tachypnea is defined as an increased respiratory rate >20 breaths per minute in adult. It is a sensitive index for the presence of occult illness in critical patients.
- Keep in mind that tachypnea is not always equal to increased work of breathing and should not be viewed as a knee jerk justification for intubation, rather it should be viewed as an expected physiologic response to lung injury or other underlying metabolic (e.g. diabetes ketoacidosis) or cardiovascular (e.g. shock state) conditions7. On the other hand increased work of breathing as evident by accessory muscle use or intercostal retraction is an ominous sign that warrants urgent action.
- 👉Hyperpnea is defined as an increase in the depth and rate of respiration leading to an increase in the minute volume of ventilation. It is consistent with an increase in metabolism as reflected by CO2 production
- 👉Hyperventilation is present (alveolar hyperventilation) when alveolar ventilation has increased out of proportion to CO2 production and the PaCO2 decreases below the normal range (<36 mmHg).
- 👉Keep in mind that both depth and duration of inspiration will affect the tidal volume.
- 👉Note that consistent increased minute ventilation causes increased work of breathing and if prolonged, respiratory fatigue and failure will be the end-result.
Perfusion
Pulmonary arteries travel besides conducting zone airways and transport deoxygenated blood from the right ventricle to alveolar capillaries via pulmonary arterioles. In turn, alveolar capillaries drain reoxygenated blood into pulmonary venules, which merge into pulmonary veins and then fill the left atrium.
Bronchial arteries are branches of the aorta (systemic circulation) and carry oxygenated blood to the respiratory parenchyma. Such blood from bronchial arteries is delivered at higher perfusion pressures than those within pulmonary arteries 5.
👉Interestingly, bronchial arterial blood also drains into the pulmonary veins, since there are no distinct bronchial veins. This mixing of venous bloods with potentially very different oxygen contents contributes to physiological shunt (figure 9).
Diffusion
Gas exchange occurs in the alveoli through a dense network of capillaries and alveoli called the alveolar–capillary network, which is assumedthe basic physiologic unit of the lung and is characterized by a large surface area and a blood supply that originates from the pulmonary arteries (figure 10.a)
The barrier between the gas in the alveoli and the red blood cells is only 1 to 2 μm in thickness and consists of type I pneumocytes, capillary endothelial cells, and their respective basement membranes. In some regions of the alveolar wall there is nothing between the airway epithelial cells and the capillary endothelial cells other than their fused basement membranes. In other regions there is a space between the epithelial and endothelial cells called the interstitial space or interstitium. The interstitium is composed of collagen, elastin, and a variety of macromolecules involved with cell–cell and cell–matrix interactions, some nerve endings, and some fibroblast-like cells.
Red blood cells pass through the pulmonary network in less than 1 second, which is sufficient time for CO2 and O2 gas exchange to occur. The binding and dissociation of O2 with Hb occurs in milliseconds, which is well suited for the average capillary transit time of 0.75 second for the red blood cell5.
The rate of diffusion of O2 and CO2 through the alveolar–capillary barrier is related to the surface area, the diffusion constant of the gas (which is related to the solubility of the gas and inversely to the square root of the molecular weight of the gas), the gas gradient across the tissue and indirectly related to the thickness of the tissue (figure 10.b). Both O2 and CO2 passively diffuse through the alveolar capillary barrier, however CO2 diffuses about 20 times more rapidly than O2.5
- Clinically in patients with lung diseases resulting in changes in diffusion (e.g. pulmonary fibrosis) there will be a decrease in PaO2 while PaCO2 may not be increased.
👉In conditions which the sympathetic tone is increased (e.g exertion, tachycardia, fever, anemia, sepsis, increased work of breathing) the transit time for red blood cells within pulmonary capillaries is shortened and the hypoxemia associated with diffusion abnormalities worsens (e.g exertional hypoxemia)5.
👉Alveolar diffusion may be enhanced by interventions like increasing the fraction of inspired O2 in the ambient air, thereby increasing the pressure gradient for oxygen between the airspace and blood that is the driving force for diffusion.
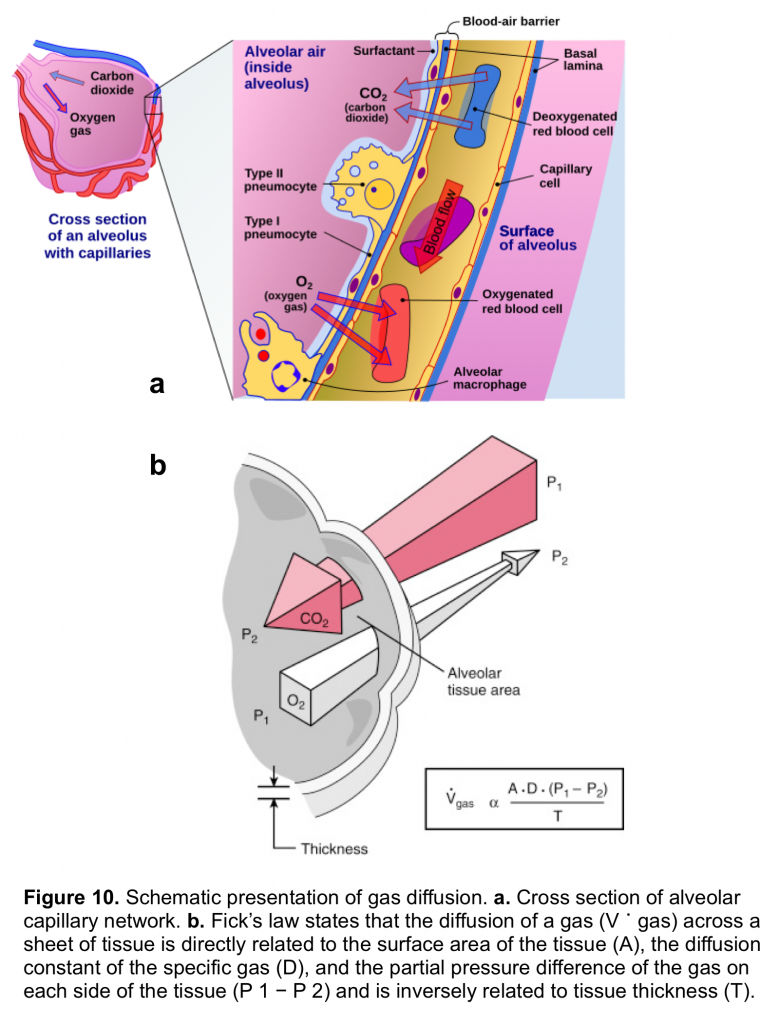
Alveolar gas equation
The alveolar gas equation can be calculated as shown below 5.
Ventilation and perfusion relationship (V/Q)
The ventilation/perfusion (V/Q) ratio is the relationship of ventilation to blood flow. It can be defined for a single alveolus, for a group of alveoli, or for the entire lung 5.
- At the level of a single alveolus, it is defined as the alveolar ventilation (VA) divided by the capillary blood flow (QC).At the level of the lung, it is defined as the total alveolar ventilation divided by the cardiac output.
In a single healthy alveolus unit, the amount of perfusion to the alveolus is well-matched to the alveolar ventilation.That is to say, a well-ventilated alveolus deserves a decent perfusion and vice versa; which makes VA/Q ≈1.
If for any good reasons the alveolus is not ventilated (e.g. mucus plug, alveolar edema, presence of pus or blood within the alveolus or atelectasia), the pulmonary arteriole is intuitively constricted directly in response to alveolar hypoxia (i.e when PAO2 is < 60mm Hg) and therefore perfusion of the poorly ventilated alveolus is diminished as flow is rather redistributed to well-ventilated lung regions (figure 12). This compensatory response (ventilation-perfusion matching) is governed by hypoxic pulmonary vasoconstriction (HPV) which diverts the blood flow away from hypoxic alveoli to improve local VA/Q matching and minimizes arterial hypoxemia for the entire lung 5.
👉In the presence of “regional” lung injury, such a compensatory response may prevail and keep arterial blood gases within normal range. However in “diffuse” lung injury, this adaptive response is inefficient and hypoxemia and/or hypercapnia develop (V/Q mismatch👇) 5.
Ventilation-perfusion mismatch (V/Q mismatch)
Ventilation-to-perfusion ratio may go wrong into two directions (figure 13). V/Q mismatch exists on a spectrum with one extreme being a shunt unit where alveolus is not ventilated at all, and a dead space unit at the other extreme; where alveolus is ventilated but not perfused (wasted ventilation)5.
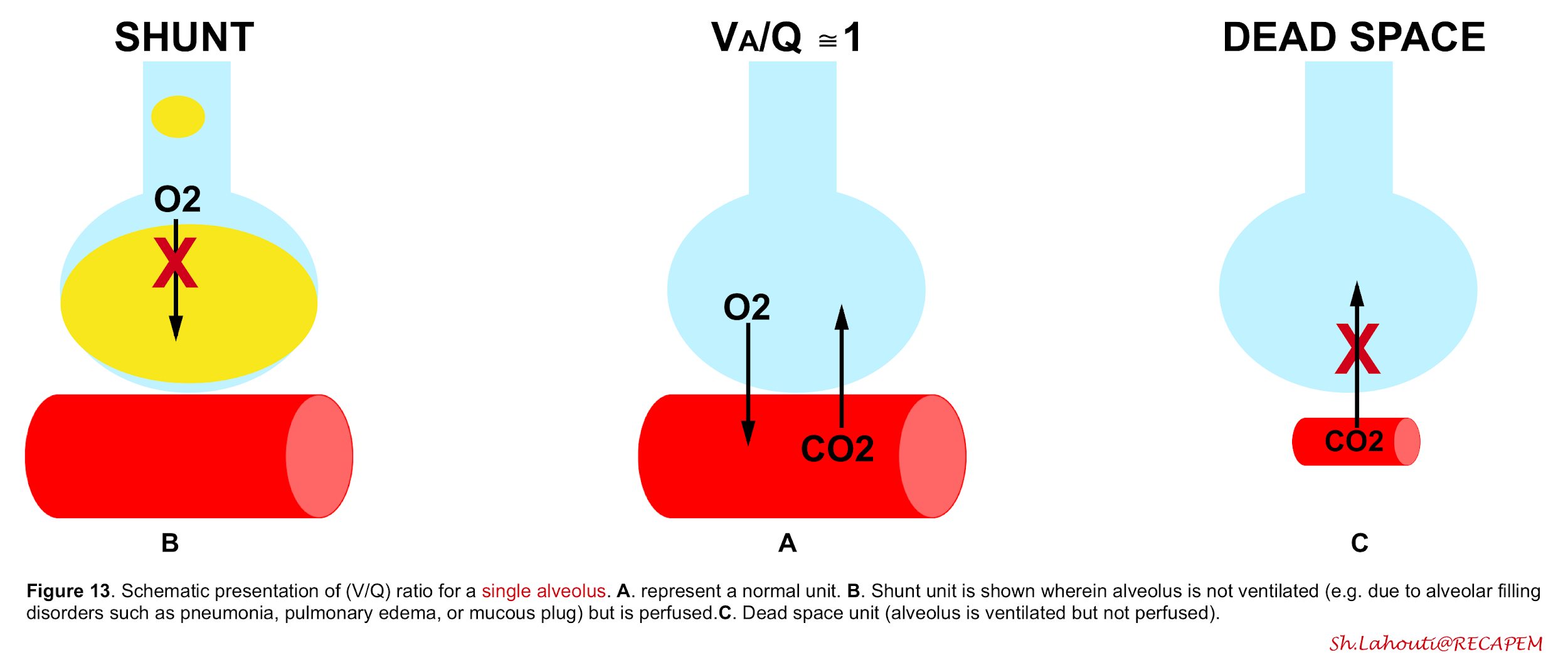
The final impact of a certain lung injury on arterial blood gases (figure 14) is determined by the nature of the disease (e.g. atelectasis which typically causes units with ↓V/Q ratio vs. massive pulmonary embolism that causes units with ↑V/Q) and the extent and severity of the disease (shunt fraction, dead space fraction).
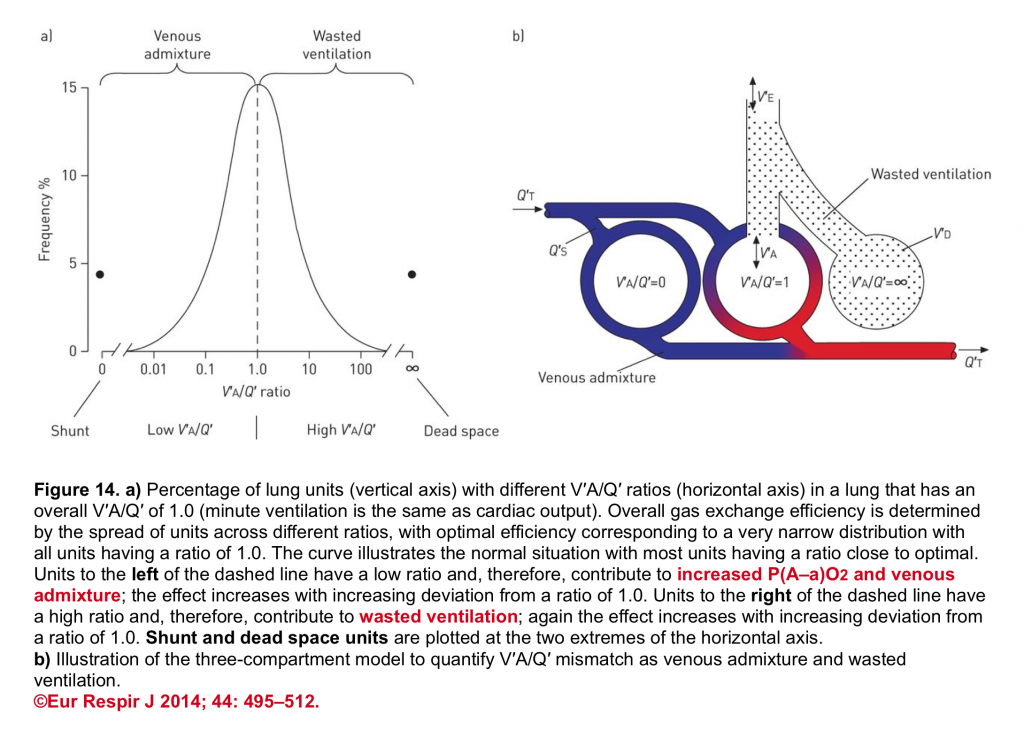
👉Keep in mind that in a certain disease, different mechanisms may operate to cause hypoxemia and/or hypercapnia.
- For example, in a patient with segmental pulmonary embolism, the lung segment with obstructed flow develops units with ↑V/Q ratio. Not uncommonly, the inflammatory mediators released from the primary involved lung region due to emboli, may spill over the neighboring segment. This inflammation can cause severe bronchospasm and contributes to atelectasis of the adjacent lung segment (unitis with ↓V/Q).
Bottom line: Any abnormality resulting in a regional alteration of either ventilation or perfusion can adversely affect pulmonary gas exchange.
- The hypoxemia associated with LOW V/Q mismatch improves with supplemental oxygen or recruitment maneuver.
- The hypercapnia associated with HIGH V/Q mismatch often improves by adjusting minute ventilation.
- 👉However shunt units induced hypoxemia does not improve to supplemental oxygenation and the hypercapnia associated with dead space units does not improve with augmenting minute ventilation either.
Oxygen transportation (Hb)
Oxygen is carried in the blood in both the dissolved gaseous state in plasma and bound to hemoglobin (Hgb) as oxyhemoglobin (HgbO2). As will be explored here,O2 transport occurs primarily through HgbO2 , with a minimal contribution of dissolved O2.
Dissolved Oxygen
- O2 diffuses passively from the alveolus to the plasma, where it dissolves. The dissolved O2 in blood is the product of the oxygen solubility times the oxygen tension and it follows a linear and nonsaturable relationship with ambient air. It is this form that is measured clinically in an ABG sample as the Pao2.
- In a healthy normal adult, each 100 mL of blood contains ≈ 0.3 mL of O2 in dissolved form.It can be seen that this amount is insufficient to meet the body’s O2 demands (the resting O2 consumption of an adult is ≈ 200-300 mL O2 /min).
Hemoglobin-bound O2
- Hb contains four iron atoms in reduced ferrous state (Fe2+),each of which can reversibly combine with one molecule of O2.The contribution of Hb to O2 transport enhances the O2-carrying capacity of blood by ≈ 65-fold.
- The principle of O2 binding to Hb differs from dissolved O2 in blood in two different ways5.
- First, oxygenation of Hb is saturable, so that once maximized, any further elevations in ambient PO2 will not increase the O2 binding capacity of Hb.
- Second, Hb binds and releases O2 in a nonlinear fashion (figure 15).
- Under optimal conditions, each Hb molecule can bind up to four O2 atoms and each gram of Hb can bind up to 1.39 mL of O2 (assuming that Hb affinity to O2 is normal and all 4 sites for O2 binding are fully saturated). This factor of 1.39 is considered the maximal O2 carrying capacity of Hb. However the Hb binding affinity to O2 is under influence of several other variables (figure 15)5.
- The total O2 content (CaO2) is the sum of the O2 bound to Hb and the dissolved O2
- 👉CaO2 = (SaO2 × Hb × 1.34 ) + ( 0.003 × PaO2 )
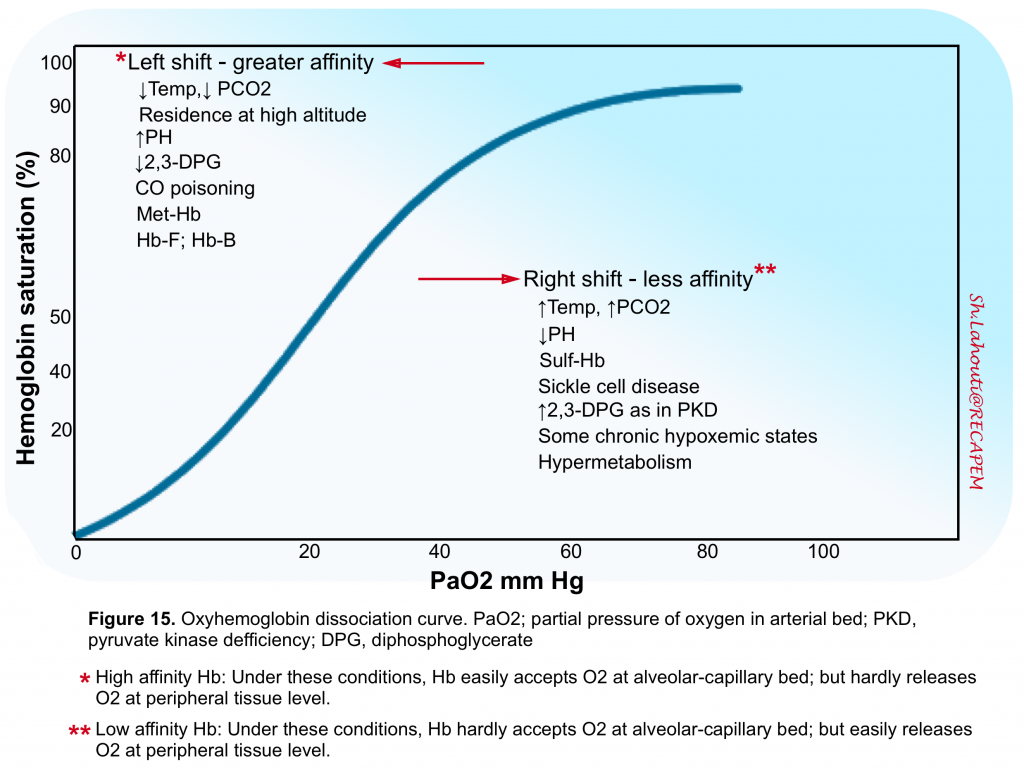
O2 Delivery (DO2)
- The amount of oxygen delivered to peripheral tissueis the result of the interplay between cardiac, pulmonary and hematologic function (figure 16). That is oxygen delivery is dependent on cardiac output and arterial oxygen content (CaO2)5.
- 👉DO2 = CO X CaO2
O2 Consumption (VO2)
- O2 is consumed by all human tissues. Global oxygen consumption (VO2) measures the total amount of oxygen consumed by the tissues per minute (figure 16)5.
- 👉VO2= CO X (CaO2 – CVO2)
- The average O2 uptake of an adult is approximately 250 mL/min, although this depends upon numerous factors 8.
O2 Extraction Ratio (O2ER)
- The amount of oxygen consumed (VO2) as a fraction of oxygen delivery (DO2) defines the oxygen extraction ratio (O2ER)
- 👉O2ER = VO2/DO2
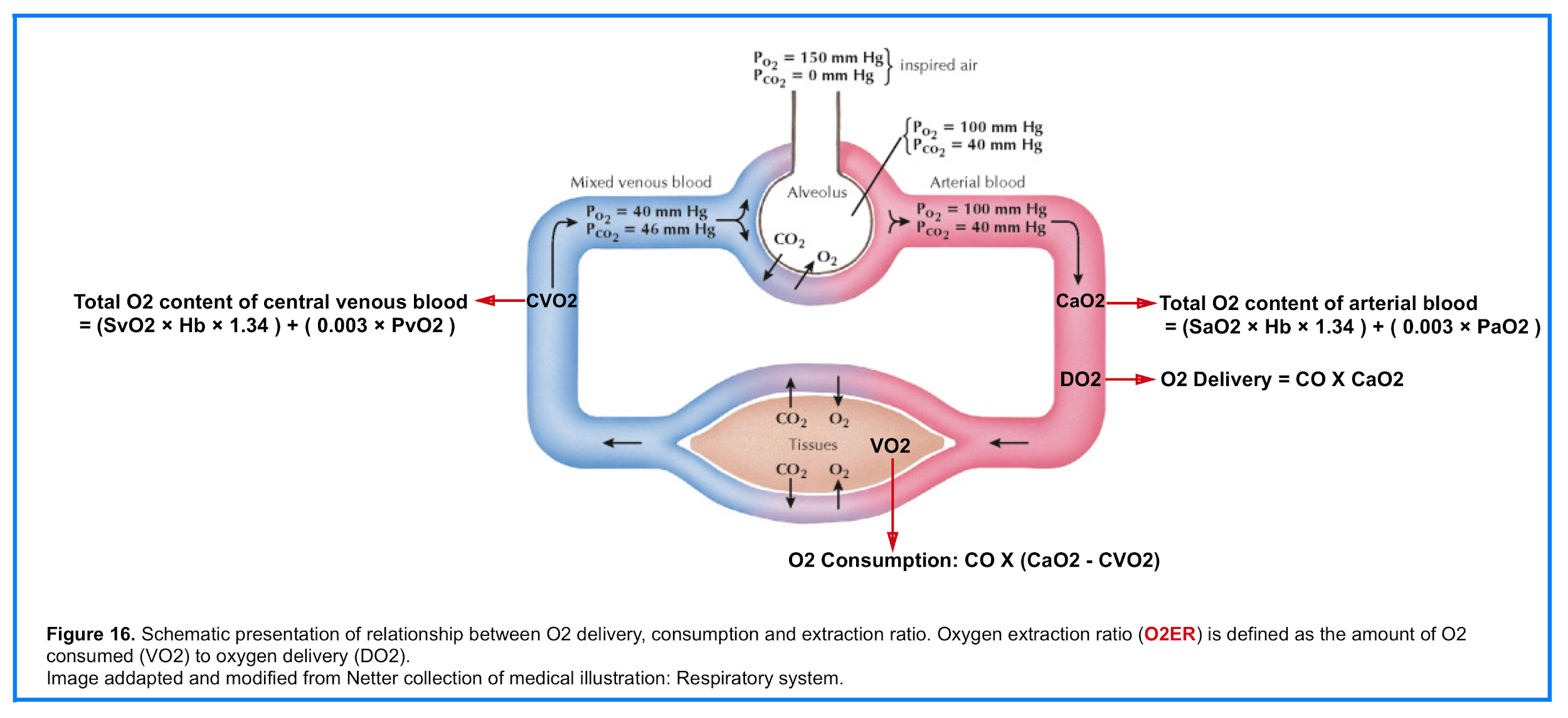
Mechanisms of tissue hypoxia
Cellular hypoxia is a state in which there is insufficient O2 to meet the metabolic demands of a given tissue. It can result from any of the following conditions:
- Hypoxemic hypoxia: Low blood O2 tension (↓PaO2)
- Anemic hypoxia: Low blood O2 content (CaO2), or abnormal Hb affinity for O2 binding
- Circulatory hypoxia: Poor perfusion to peripheral tissue (ischemia)
- Histologic hypoxia: refers to block in electron transport in mitochondrial respiration (e.g. carbon monoxide or cyanide poisoning etc)5 9
Pathophysiology and etiology of hypoxemia
Hypoxemia
- It is an abnormally low arterial oxygen tension (defined as a PaO2 <60 mm Hg) and results from any combination of 5 mechanisms listed in the following table (figure 17)5 10.
Relative hypoxemia
- It is the term used when the arterial oxygen tension is lower than expected for a given level of inhaled oxygen which reflects inefficient O2 transfer from the lungs to the circulation.
- The degree of relative hypoxemia can be assessed by calculating the alveolar–arterial (A-a) oxygen partial pressure gradient, P(A-a)O2. Calculation of PAO2 is explained above (figure 11). PaO2 is displayed by arterial blood gas analysis.
- A normal P(A-a) O2 is <10 mm Hg in young, healthy patients and increases with age, predicted by the formula as: P(A-a)O2 = 2.5 + (0.2 x age in years)
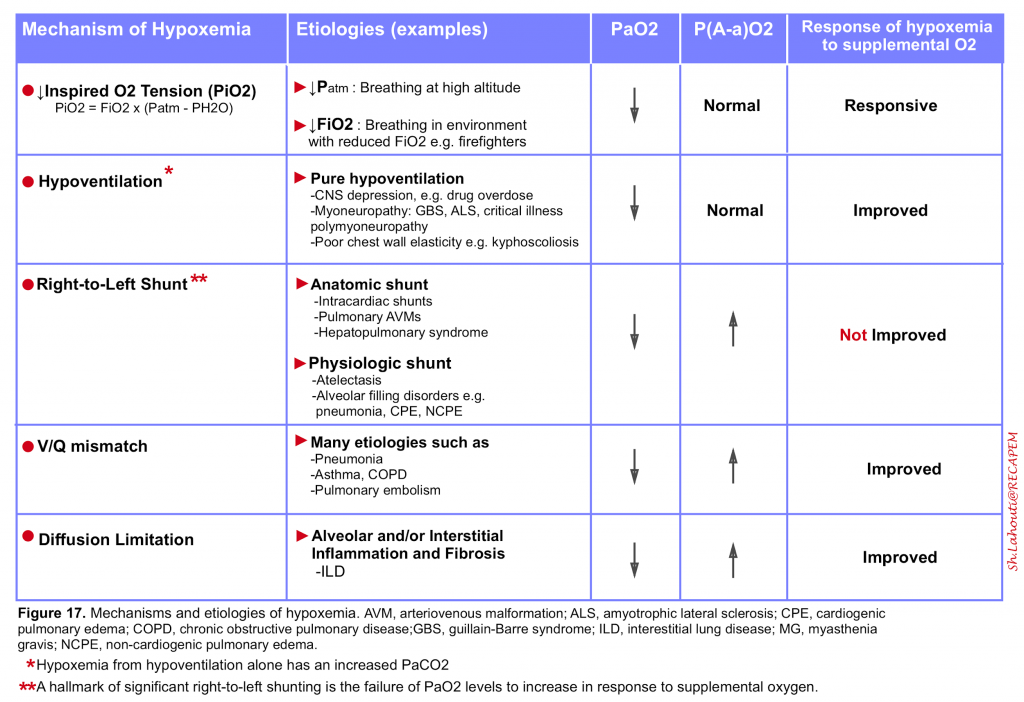
Pathophysiology and etiology of hypercapnia
It is defined as a PaCO2 >45 mm Hg and results from alveolar hypoventilation either as a result of “global hypoventilation” or increased wasted ventilation (dead space) 5. The mechanism and etiologies of hypercapnia is listed in the following table (figure 19)11. Anatomic dead space (figure 8) and alveolar dead space (figure 13) have been discussed above.
- It is worth mentioning that increased CO2 production alone can rarely cause hypercapnia unless it is accompanied by respiratory muscle fatigue.
PETCO2
- Recent studies have shown that measurement of end-tidal CO2 (PETCO2)via capnogram can be helpful in identification of alveolar dead space ventilation.
- At expiration, the first part of the exhaled gas originates from the anatomical dead space where no gas exchange occurs.
- Therefore, this gas has the same PCO2 as inspired gas, usually close to zero (figure 18).
- After a short transitional phase consisting of a mixture of anatomical dead space and alveolar gas, the rest of the expiration consists of mixed alveolar gas.
- Measurement of PCO2 of exhaled gas at the end of the expiration, PETCO2, has been used as a measure of mixed alveolar gas and as a noninvasive measure of PaCO2 (figure 18)5.
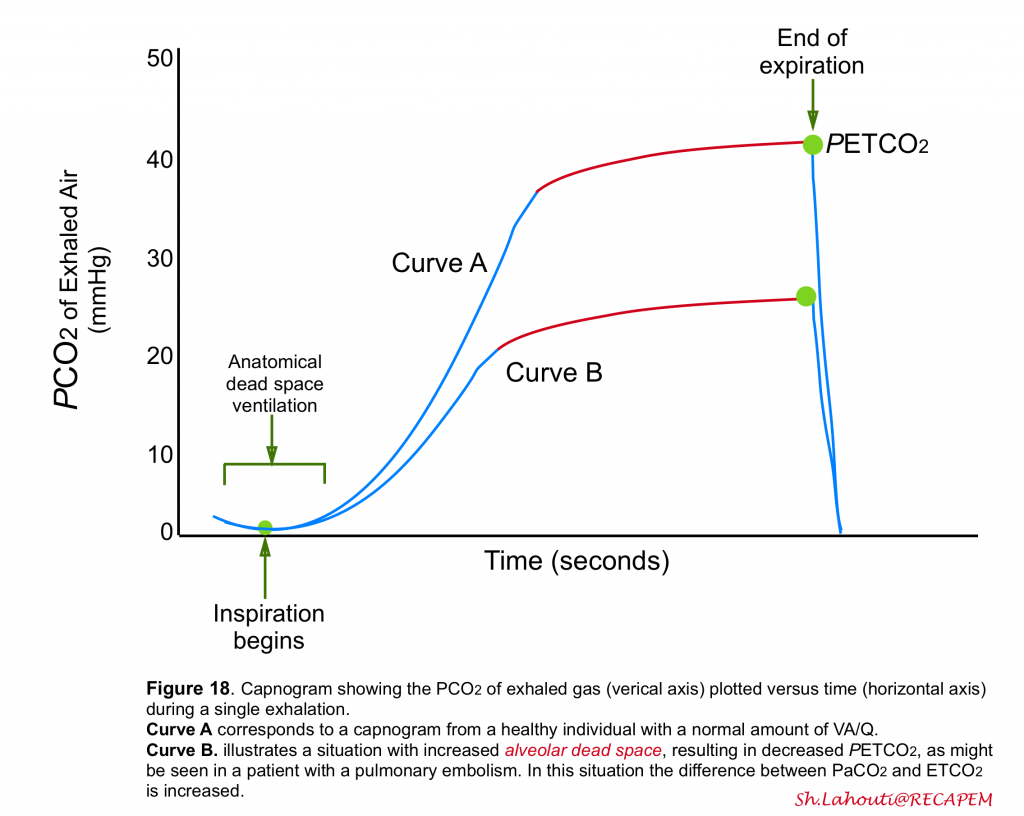
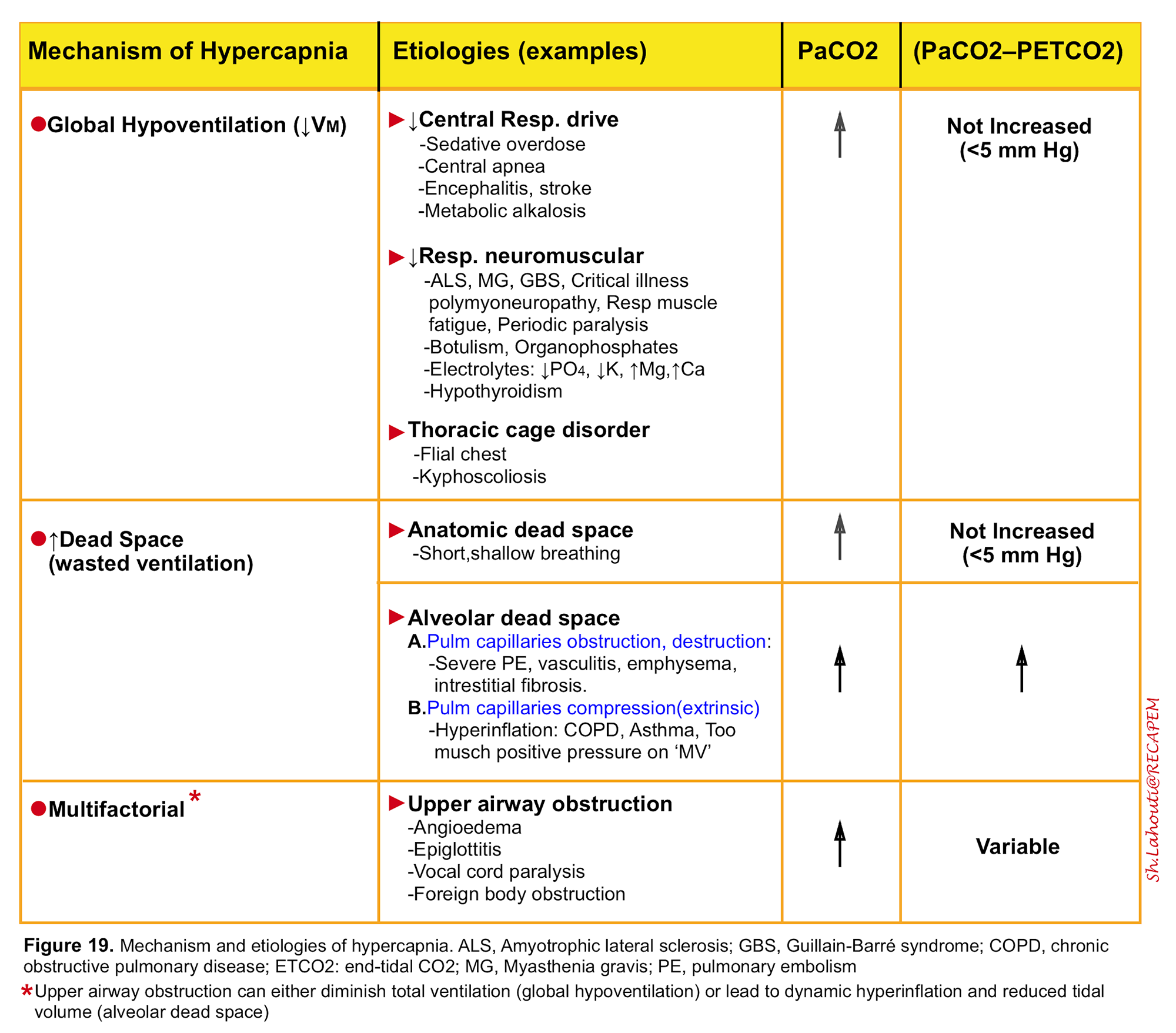
Respiratory distress syndrome
Clinical Presentation
The spectrum of severity of respiratory distress syndrome and associated sings and symptoms are presented below.
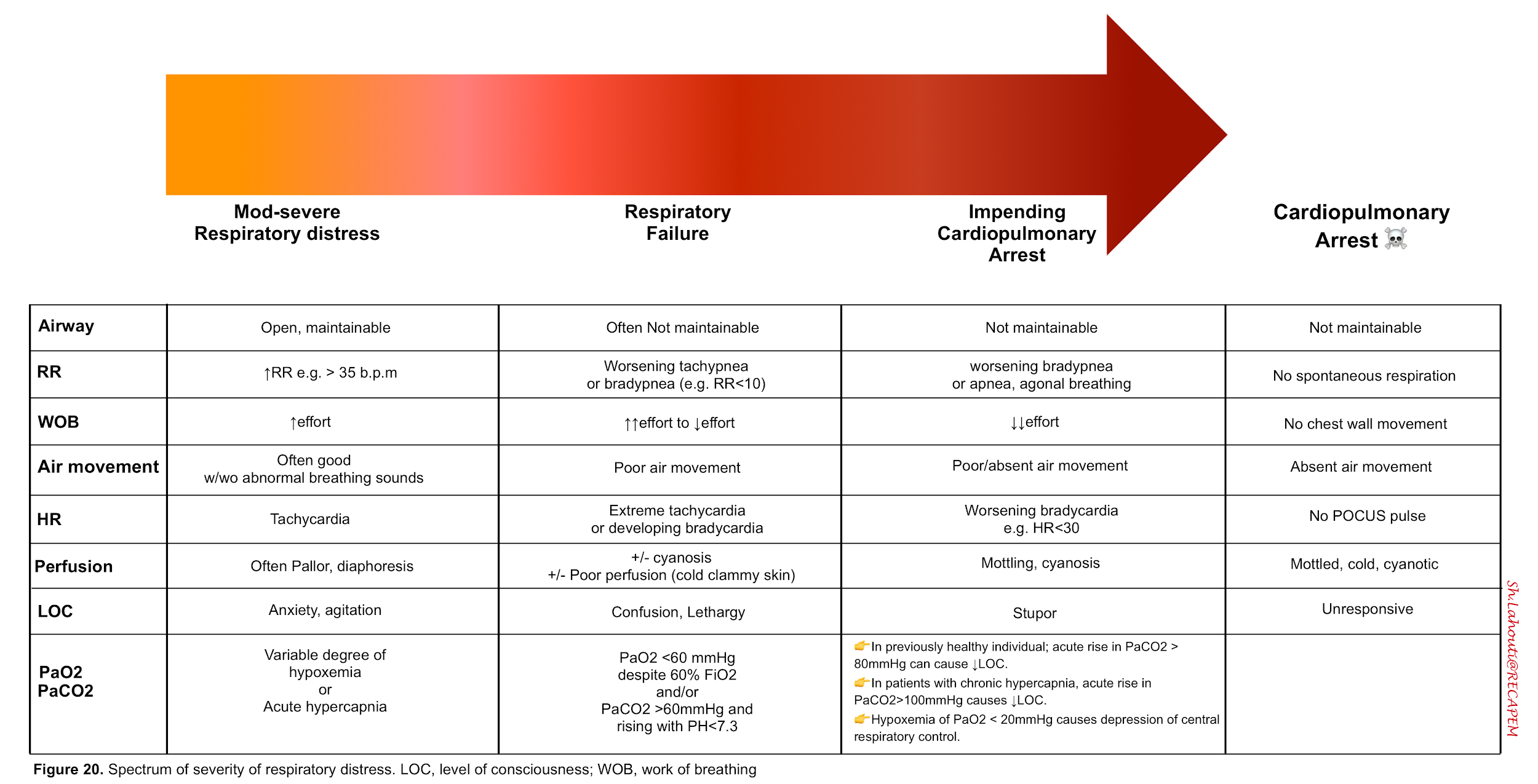
Symptoms
- Dyspnea
- It is defined as a subjective sense of breathing discomfort that its quality and intensity varies among patients12. Physicians should assess acuity, quality and severity of dyspnea and consider a broad differential diagnosis while simultaneously initiating potentially life saving treatments.
- Generally acute onset and severe dyspnea (e.g. unable to complete a sentence; breathless while dressing) is more worrisome.
- Never ignore undifferentiated dyspnea. It has been shown that shortness of the breath is a harbinger of increased mortality.
- Dyspnea is a feature of several disorders shown in the table below (figure 24)13.
- Orthopnea
- Refers to dyspnea in the supine position and is most often due to left ventricular failure and less commonly can be seen in patients with diaphragmatic paralysis, chronic obstructive pulmonary disease and central obesity.
- Patients with decompensated heart failure may report worsening dyspnea upon bending forward (bendopnea) 44 .
- Paroxysmal nocturnal dyspnea (PND)
- It is orthopnea that awakens the patient from sleep, prompting an upright posture in order to resolve breathlessness. It is a more specific (84%) symptom for decompensated heart failure45 .
- Platypnea
- Refers to dyspnea in the upright position (the opposite of orthopnea). Platypnea can be seen in patients with “hepatopulmonary syndrome” (HPS) 46 or those with loss of abdominal wall muscular tone. It is also rarely seen in patients with intracardiac right-to-left shunting (e.g. patent foramen ovale).
Physical findings on exam
- Signs of increased work of breathing
- Worsening tachypnea (>35 b.p.m) suggests that the patient may eventually tire out .
- Use of the accessory respiratory muscles (sternocleidomastoid, sternoclavicular, and intercostals)14.👉Keep in mind that patients with neuromuscular disease may not demonstrate accessory muscle use despite presence of severe respiratory compromise.
- Shallow breathing
- Tripoding
- Paradoxical abdominal wall movement when the abdominal wall retracts inward with inspiration, indicating diaphragmatic fatigue.
- Diaphoresis reflects extreme sympathetic response associated with severe disease
- Cyanosis
- Defined as bluish discoloration of skin and mucous membrane as a result of increased “absolute” amount of deoxy-Hb (deoxy-Hb >5 g/dL; which roughly corresponds to SaO2 of 67%) or hemoglobin derivatives15.
- Central cyanosis is detected at mucous membranes and tongue, and is due to inadequate pulmonary oxygenation (see etiology of hypoxemia, figure 17) or an abnormal hemoglobin (e.g Methemoglobin or sulfhemoglobin).
- Peripheral cyanosis is detected at fingers or extremities and is the result of vasoconstriction or poor perfusion (e.g shock states).
- All conditions that cause central cyanosis eventually result in peripheral cyanosis as well.
- In addition to “absolute” amounts of deoxy-Hb or hemoglobin derivatives; several other physiologic and anatomic factors are influential in “physical appearance” of cyanosis.
- 👉Therefore detection of cyanosis is neither sensitive nor a specific indicator of the state of arterial oxygenation.
- 👉Patients with certain congenital heart disease (Eisenmenger syndrome) have chronic cyanosis at their baseline.
- Defined as bluish discoloration of skin and mucous membrane as a result of increased “absolute” amount of deoxy-Hb (deoxy-Hb >5 g/dL; which roughly corresponds to SaO2 of 67%) or hemoglobin derivatives15.
- Associated signs and symptoms
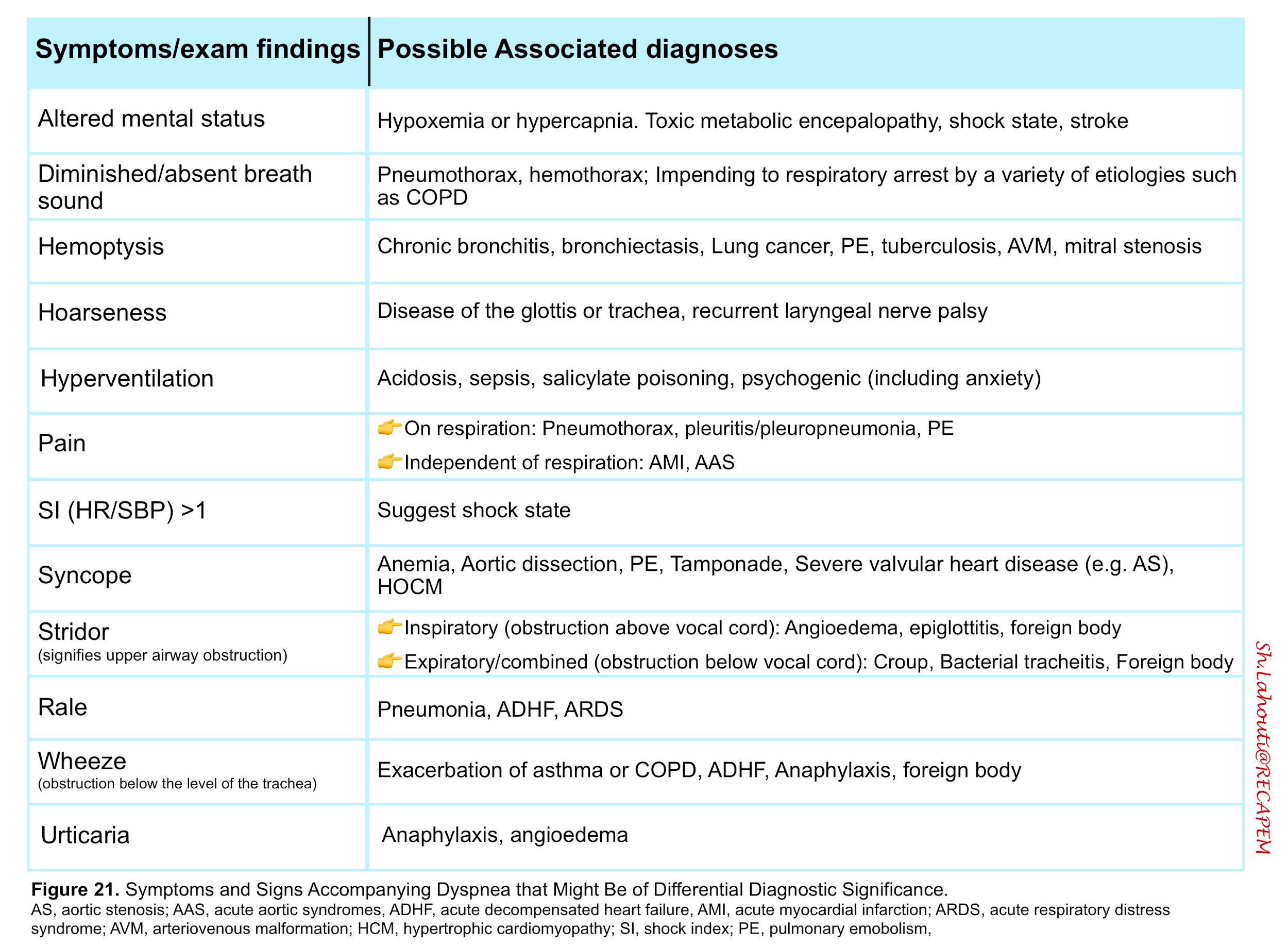
Considerations
It’s worthy to review some signs and symptoms related to hypoxemia and hypercapnia here.
Hypoxemia
- Clinical manifestation of tissue hypoxia may include but not limited to agitation, obtundation, seizure, coma. However an abnormal SaO2 below which tissue hypoxia develops has not been clearly defined. This reflects the multifactorial nature of tissue hypoxia which has already been discussed (i.e. hypoxemia does not always lead to tissue hypoxia as long as O2 delivery and tissue O2 extraction is compensated for the level of hypoxemia).
- Hypoxemia (via chemoreceptors) drives respiratory adaptive responses. The resulting tachypnea and hyperventilation may correct arterial hypoxemia. However at PaO2 of < 20 mm Hg, there is a central depression of respiration.
- Hypoxemia evokes other adaptive responses as well. For example O2 delivery is augmented via increasing cardiac output (as by increasing heart rate). However in patients with severe respiratory distress impending to cardiorespiratory arrest, compensatory mechanisms fail and bradycardia ensues.
- In general, healthy individuals demonstrate SaO2 of 95% or greater. Older adults and heavy smokers often maintain levels between 92-95%. Patients with chronic lung disease may have baseline level below 92%.
- The values of SaO2 should not be interpreted in isolation.
- In the presence of other cardiorespiratory signs and symptoms such as dyspnea, always consider patient’s associated comorbidities and baseline SaO2.
Hypercapnia
- The clinical signs of hypercapnia are mostly neurologic and pulmonary and vary depending on the absolute value of PaCO2 and its rate of change 16.
- Normal individuals do not develop a depressed level of consciousness until the PaCO2 is > 80 mm Hg.
- In contrast patients with chronic hypercapnia (e.g COPD) may not develop symptoms until PaCO2 is >100 mm Hg.
- While mild-moderate hypercapnia drives the respiratory center, critical hypercapnia has a sedative effect on the central nervous system and patients develop lethargy, coma (CO2 narcosis).
- Mild slowly developing hypercapnia can cause headache, anxiety, daytime sleepiness and dyspnea.
- Patients with critical hypercapnia may present with asterixis, myoclonus, seizures, papilledema, and progressive deterioration of level of consciousness as well as cardiovascular collapse.
- ☠️ In patients with hypoxic respiratory distress, development of hypercapnia with respiratory acidosis is an ominous sign implying impending respiratory arrest.
- 👉In acute or acute-on-chronic respiratory acidosis, the PaCO2 is elevated above the upper limit of the reference range with an accompanying acidemia (i.e. pH <7.30), while In chronic compensated respiratory acidosis, the PaCO2 is elevated, but the pH is at the lower limit of normal or near-normal pH (eg, pH 7.30 to 7.35).
- However these rules may be influenced by presence of another coexistent acid-base disturbance and a systematic approach to interpretation of ‘VBG’ is recommended.
Bedside Ultrasound
Bedside lung US can differentiate acute decompensated heart failure from noncardiac causes of acute dyspnea.
- Bedside US can also identify pleural effusion, pneumothorax, cardiac tamponade, cardiac functional abnormalities, pulmonary consolidation, and intravascular volume status17.
- Several protocols have been proposed for ultrasound evaluation of adult patients with respiratory distress (e.g. BLUE protocol 18 19, Search 8Es 20) and lung ultrasound in hemodynamic assessment of shock patients (e.g. FALLS protocol 21).
- However in the light of recent guidelines regarding diagnosis of pulmonary embolism 22,Right heart failure 23 24,pulmonary hypertension 25 and recommendation from american society of echocardiography (ASE) and European society of cardiology (ESC) for evaluation of adult with diastolic 26 and systolic 27 28 dysfunction; we propose the following protocol for evaluation of adult patients with respiratory distress which requires further validation (figure 23).
Differential Diagnosis
Although the differential diagnosis for tachypnea and dyspnea are broad (figure 24) 13, not all patients meet the definition of respiratory distress and failure.
- The most common diagnoses in older adult patients with acute dyspnea are acute decompensated heart failure, pneumonia, chronic obstructive pulmonary disease (COPD), pulmonary embolism, and asthma 31.
Tips
- 👉Have eagle eyes and consider a broad differential diagnosis to avoid missed or delayed diagnosis and/or treatment.” Do not narrow down your differentials so quickly.
- 👉Keep in mind that patients may present with more than one cause of respiratory distress such as “acute decompensated heart failure PLUS Pneumonia”.
For more on ARDS👉 here.
Approach to Diagnosis and Management
General principles of diagnosis
- The cause of dyspnea in most ED patients can be identified by the history, physical examination, ECG and POCUS 47.
- For every patient presenting with acute dyspnea in the ED, the following assessment for impending respiratory failure or cardiovascular collapse should be performed while simultaneously resucitation measures should be undertaken.
Tips: Keep in mind the pitfalls of pulse oximetry.
- First, It may not be accurate in the setting of hypothermia, shock, carbon monoxide poisoning, and methemoglobinemia.
- Second, It takes seconds to minutes for the SaO2 to reflect treatment changes for arterial hypoxemia (e.g. increase or decrease of supplemental oxygen). Longer response times are expected if there is reduced cardiac output. This delay should be considered when making decisions regarding the escalation and de-escalation of oxygen therapy.
Tips: 🚩Keep in mind the Red flags for dyspnea
- Dyspnea developing over minutes to hours
- Difficulty speaking in full sentence
- HR > 120
- RR > 30 b.p.m
- Oxygen saturation < 90%
- Accessory respiratory muscle use
- Diaphoresis
- Cyanosis
- Stridor, asymmetric breath sound, diffuse crackle
Principles of management
For every patient presenting with acute dyspnea in the ED, the following basic resuscitative measures should be performed
- Position the patient in best comfortable way to enhance breathing.
- IV access
- Provide oxygen to maintain adequate SaO2 ~94% 32
- Patients who are not hypoxemic have not been shown to benefit from, and may be harmed by, the administration of supplemental oxygen 33.
- Do not withhold oxygen required to maintain minimum oxygen saturation levels in any chronic lung disease patient (e.g. COPD) in an effort to stimulate ventilation and reduce hypercapnia 48 49.
- The management strategies to support oxygenation and ventilation as well airway management are summarized below.
Tips: Treat the patient but not the numbers!
- SaO2 of 98% is not reassuring if your patient has signs of increased work of breathing as this may indicate acute severe hypercapnia and pulse oximetry reading is irrelevant.
- 👉For the same token, patients with COPD often have high baseline PaCO2. Always check gas values with the patient’s baseline and clinical status.
- More on non-invasive ventilation, here .
- More on airway management here.
- For COVID airway management here
Specific therapeutic measures
Respiratory distress syndrome can be caused by a broad list of diseases. Each clinical condition warrants specific therapeutic measures. The following table summarizes treatment plan for common causes of respiratory distress.
Appendix
Signs and symptoms of COVID-19
Prognostic value and cutoff levels of imaging parameters
Going further
- Dyspnea approach and differential (EM:RAP)
- Dyspnea: Management (EM:RAP)
- Respiratory distress: An emergency medicine approach (First10EM)
References
1. Treacher DF, Leach RM. Oxygen transport-1. Basic principles. BMJ. 1998;317(7168):1302-1306. doi:10.1136/bmj.317.7168.1302
Hsia CC, Schmitz A, Lambertz M, Perry SF, Maina JN. Evolution of air breathing: oxygen homeostasis and the transitions from water to land and sky. Compr Physiol. 2013;3(2):849-915. doi:10.1002/cphy.c120003
2. Roussos C, Koutsoukou A. Respiratory failure. Eur Respir J Suppl. 2003 Nov;47:3s-14s. doi: 10.1183/09031936.03.00038503. PMID: 14621112
3. Schwartzstein RM, and Lewis A. Chapter 29: Dyspnea. In: Broaddus V, Mason RC, and Ernst JD, eds. Murray & Nadel’s textbook of respiratory medicine. 6th edition. Philadelphia, PA: Elsevier Health Sciences, Saunders/Elsevier; 2015:490-491.
4. Petersson J, Glenny RW. Gas exchange and ventilation-perfusion relationships in the lung. Eur Respir J. 2014 Oct;44(4):1023-41. doi: 10.1183/09031936.00037014. Epub 2014 Jul 25. PMID: 25063240
5. Cameron, J., & Misra, U. (2017). Respiration: ventilation. Anaesthesia and Intensive Care Medicine, 18(12), 634–638. https://doi.org/10.1016/j.mpaic.2017.09.012
6. Tobin MJ. Basing Respiratory Management of COVID-19 on Physiological Principles. Am J Respir Crit Care Med. 2020 Jun 1;201(11):1319-1320. doi: 10.1164/rccm.202004-1076ED. PMID: 32281885; PMCID: PMC7258630
7. Leach RM, Treacher DF. The pulmonary physician in critical care * 2: oxygen delivery and consumption in the critically ill. Thorax. 2002;57(2):170-177. doi:10.1136/thorax.57.2.170
8. Leach RM, Treacher DF. The pulmonary physician in critical care * 2: oxygen delivery and consumption in the critically ill. Thorax. 2002;57(2):170-177. doi:10.1136/thorax.57.2.170
9. Rodríguez-Roisin R, Roca J. Mechanisms of hypoxemia. Intensive Care Med. 2005 Aug;31(8):1017-9. doi: 10.1007/s00134-005-2678-1. Epub 2005 Jun 14. PMID: 16052273
10. Masterson C, Otulakowski G, Kavanagh BP. Hypercapnia: clinical relevance and mechanisms of action. Curr Opin Crit Care. 2015 Feb;21(1):7-12. doi: 10.1097/MCC.0000000000000164. PMID: 25546531
11. Parshall MB, Schwartzstein RM, Adams L, Banzett RB, Manning HL, Bourbeau J, Calverley PM, Gift AG, Harver A, Lareau SC, Mahler DA, Meek PM, O’Donnell DE; American Thoracic Society Committee on Dyspnea. An official American Thoracic Society statement: update on the mechanisms, assessment, and management of dyspnea. Am J Respir Crit Care Med. 2012 Feb 15;185(4):435-52. doi: 10.1164/rccm.201111-2042ST. PMID: 22336677; PMCID: PMC5448624
12. Berliner D, Schneider N, Welte T, Bauersachs J. The Differential Diagnosis of Dyspnea. Dtsch Arztebl Int. 2016 Dec 9;113(49):834-845. doi: 10.3238/arztebl.2016.0834. PMID: 28098068; PMCID: PMC5247680
13. Tulaimat A, Patel A, Wisniewski M, Gueret R. The validity and reliability of the clinical assessment of increased work of breathing in acutely ill patients. J Crit Care. 2016 Aug;34:111-5. doi: 10.1016/j.jcrc.2016.04.013. Epub 2016 Apr 27. PMID: 27288621
14. McMullen, S. M., & Patrick, W. (2013). Cyanosis. American Journal of Medicine, 126(3), 210–212. https://doi.org/10.1016/j.amjmed.2012.11.004
15. Davidson AC, Banham S, Elliott M, Kennedy D, Gelder C, Glossop A, Church AC, Creagh-Brown B, Dodd JW, Felton T, Foëx B, Mansfield L, McDonnell L, Parker R, Patterson CM, Sovani M, Thomas L; BTS Standards of Care Committee Member, British Thoracic Society/Intensive Care Society Acute Hypercapnic Respiratory Failure Guideline Development Group, On behalf of the British Thoracic Society Standards of Care Committee. BTS/ICS guideline for the ventilatory management of acute hypercapnic respiratory failure in adults. Thorax. 2016 Apr;71 Suppl 2:ii1-35. doi: 10.1136/thoraxjnl-2015-208209. Erratum in: Thorax. 2017 Jun;72 (6):588. PMID: 26976648
16. Dempsey, A. F., & Mehl, J. (2016). Critical Care Ultrasound. In Anesthesia & Analgesia (Vol. 123, Issue 1). https://doi.org/10.1213/ane.0000000000001292
17. Bekgoz B, Kilicaslan I, Bildik F, Keles A, Demircan A, Akoglu O, Coskun G, Demir HA. BLUE protocol ultrasonography in Emergency Department patients presenting with acute dyspnea. Am J Emerg Med. 2019 Nov;37(11):2020-2027. doi: 10.1016/j.ajem.2019.02.028. Epub 2019 Feb 20. PMID: 30819579
18. Lichtenstein D. Novel approaches to ultrasonography of the lung and pleural space: where are we now? Breathe (Sheff). 2017 Jun;13(2):100-111. doi: 10.1183/20734735.004717. PMID: 28620429; PMCID: PMC5467658
19. Ahn JH, Jeon J, Toh HC, Noble VE, Kim JS, Kim YS, Do HH, Ha YR. SEARCH 8Es: A novel point of care ultrasound protocol for patients with chest pain, dyspnea or symptomatic hypotension in the emergency department. PLoS One. 2017 Mar 29;12(3):e0174581. doi: 10.1371/journal.pone.0174581. PMID: 28355246; PMCID: PMC5371336
20. Lichtenstein D. FALLS-protocol: lung ultrasound in hemodynamic assessment of shock. Heart Lung Vessel. 2013;5(3):142-147.
Konstantinides SV, Meyer G, Becattini C, Bueno H, Geersing GJ, Harjola VP, Huisman MV, Humbert M, Jennings CS, Jiménez D, Kucher N, Lang IM, Lankeit M, Lorusso R, Mazzolai L, Meneveau N, Ní Áinle F, Prandoni P, Pruszczyk P, Righini M, Torbicki A, Van Belle E, Zamorano JL; ESC Scientific Document Group. 2019 ESC Guidelines for the diagnosis and management of acute pulmonary embolism developed in collaboration with the European Respiratory Society (ERS). Eur Heart J. 2020 Jan 21;41(4):543-603. doi: 10.1093/eurheartj/ehz405. PMID: 31504429.
21. Harjola VP, Mebazaa A, Čelutkienė J, Bettex D, Bueno H, Chioncel O, Crespo-Leiro MG, Falk V, Filippatos G, Gibbs S, Leite-Moreira A, Lassus J, Masip J, Mueller C, Mullens W, Naeije R, Noordegraaf AV, Parissis J, Riley JP, Ristic A, Rosano G, Rudiger A, Ruschitzka F, Seferovic P, Sztrymf B, Vieillard-Baron A, Yilmaz MB, Konstantinides S. Contemporary management of acute right ventricular failure: a statement from the Heart Failure Association and the Working Group on Pulmonary Circulation and Right Ventricular Function of the European Society of Cardiology. Eur J Heart Fail. 2016 Mar;18(3):226-41. doi: 10.1002/ejhf.478. PMID: 26995592
22. Rudski LG, Lai WW, Afilalo J, Hua L, Handschumacher MD, Chandrasekaran K, Solomon SD, Louie EK, Schiller NB. Guidelines for the echocardiographic assessment of the right heart in adults: a report from the American Society of Echocardiography endorsed by the European Association of Echocardiography, a registered branch of the European Society of Cardiology, and the Canadian Society of Echocardiography. J Am Soc Echocardiogr. 2010 Jul;23(7):685-713; quiz 786-8. doi: 10.1016/j.echo.2010.05.010. PMID: 20620859.
23. Galiè N, Humbert M, Vachiery JL, Gibbs S, Lang I, Torbicki A, Simonneau G, Peacock A, Vonk Noordegraaf A, Beghetti M, Ghofrani A, Gomez Sanchez MA, Hansmann G, Klepetko W, Lancellotti P, Matucci M, McDonagh T, Pierard LA, Trindade PT, Zompatori M, Hoeper M; ESC Scientific Document Group. 2015 ESC/ERS Guidelines for the diagnosis and treatment of pulmonary hypertension: The Joint Task Force for the Diagnosis and Treatment of Pulmonary Hypertension of the European Society of Cardiology (ESC) and the European Respiratory Society (ERS): Endorsed by: Association for European Paediatric and Congenital Cardiology (AEPC), International Society for Heart and Lung Transplantation (ISHLT). Eur Heart J. 2016 Jan 1;37(1):67-119. doi: 10.1093/eurheartj/ehv317. Epub 2015 Aug 29. PMID: 26320113.
24. Nagueh SF, Smiseth OA, Appleton CP, Byrd BF 3rd, Dokainish H, Edvardsen T, Flachskampf FA, Gillebert TC, Klein AL, Lancellotti P, Marino P, Oh JK, Popescu BA, Waggoner AD. Recommendations for the Evaluation of Left Ventricular Diastolic Function by Echocardiography: An Update from the American Society of Echocardiography and the European Association of Cardiovascular Imaging. J Am Soc Echocardiogr. 2016 Apr;29(4):277-314. doi: 10.1016/j.echo.2016.01.011. PMID: 27037982
25. Hu K, Liu D, Herrmann S, Niemann M, Gaudron PD, Voelker W, Ertl G, Bijnens B, Weidemann F. Clinical implication of mitral annular plane systolic excursion for patients with cardiovascular disease. Eur Heart J Cardiovasc Imaging. 2013 Mar;14(3):205-12. doi: 10.1093/ehjci/jes240. Epub 2012 Nov 15. PMID: 23161791
26. Matos J, Kronzon I, Panagopoulos G, Perk G. Mitral annular plane systolic excursion as a surrogate for left ventricular ejection fraction. J Am Soc Echocardiogr. 2012 Sep;25(9):969-74. doi: 10.1016/j.echo.2012.06.011. Epub 2012 Jul 12. PMID: 22795199
27. Hernandez-Suarez DF, Lopez-Menendez F, Roche-Lima A, Lopez-Candales A. Assessment of Mitral Annular Plane Systolic Excursion in Patients With Left Ventricular Diastolic Dysfunction. Cardiol Res. 2019 Apr;10(2):83-88. doi: 10.14740/cr837. Epub 2019 Apr 11. PMID: 31019637; PMCID: PMC6469911
28. Bergenzaun L, Ohlin H, Gudmundsson P, Willenheimer R, Chew MS. Mitral annular plane systolic excursion (MAPSE) in shock: a valuable echocardiographic parameter in intensive care patients. Cardiovasc Ultrasound. 2013 May 30;11:16. doi: 10.1186/1476-7120-11-16. PMID: 23718803; PMCID: PMC3679845
29. Ray P, Biroleau S, Lefort Y, Becquemin MH, Beigelman C, Isnard R, Teixeira A, Arthaud M, Riou B, Boddaert J. Acute respiratory failure in the elderly: etiology, emergency diagnosis and prognosis. Crit Care. 2006;10(3):R82. doi: 10.1186/cc4926. Epub 2006 May 24. PMID: 16723034; PMCID: PMC1550946.
30. O’Driscoll BR, Howard LS, Earis J, Mak V; British Thoracic Society Emergency Oxygen Guideline Group; BTS Emergency Oxygen Guideline Development Group. BTS guideline for oxygen use in adults in healthcare and emergency settings. Thorax. 2017 Jun;72(Suppl 1):ii1-ii90. doi: 10.1136/thoraxjnl-2016-209729. PMID: 28507176
31. Chu DK, Kim LH, Young PJ, Zamiri N, Almenawer SA, Jaeschke R, Szczeklik W, Schünemann HJ, Neary JD, Alhazzani W. Mortality and morbidity in acutely ill adults treated with liberal versus conservative oxygen therapy (IOTA): a systematic review and meta-analysis. Lancet. 2018 Apr 28;391(10131):1693-1705. doi: 10.1016/S0140-6736(18)30479-3. Epub 2018 Apr 26. PMID: 29726345
32. Frat JP, Thille AW, Mercat A, Girault C, Ragot S, Perbet S, Prat G, Boulain T, Morawiec E, Cottereau A, Devaquet J, Nseir S, Razazi K, Mira JP, Argaud L, Chakarian JC, Ricard JD, Wittebole X, Chevalier S, Herbland A, Fartoukh M, Constantin JM, Tonnelier JM, Pierrot M, Mathonnet A, Béduneau G, Delétage-Métreau C, Richard JC, Brochard L, Robert R; FLORALI Study Group; REVA Network. High-flow oxygen through the nasal cannula in acute hypoxemic respiratory failure. N Engl J Med. 2015 Jun 4;372(23):2185-96. doi: 10.1056/NEJMoa1503326. Epub 2015 May 17. PMID: 25981908
33. Miguel-Montanes R, Hajage D, Messika J, Bertrand F, Gaudry S, Rafat C, Labbé V, Dufour N, Jean-Baptiste S, Bedet A, Dreyfuss D, Ricard JD. Use of high-flow nasal cannula oxygen therapy to prevent desaturation during tracheal intubation of intensive care patients with mild-to-moderate hypoxemia. Crit Care Med. 2015 Mar;43(3):574-83. doi: 10.1097/CCM.0000000000000743. PMID: 25479117
Kelly CR, Higgins AR, Chandra S. Noninvasive Positive-Pressure Ventilation. N Engl J Med. 2015 Sep 24;373(13):1279. doi: 10.1056/NEJMc1508384. PMID: 26398090
34. Rochwerg B, Brochard L, Elliott MW, Hess D, Hill NS, Nava S, Navalesi P Members Of The Steering Committee, Antonelli M, Brozek J, Conti G, Ferrer M, Guntupalli K, Jaber S, Keenan S, Mancebo J, Mehta S, Raoof S Members Of The Task Force. Official ERS/ATS clinical practice guidelines: noninvasive ventilation for acute respiratory failure. Eur Respir J. 2017 Aug 31;50(2):1602426. doi: 10.1183/13993003.02426-2016. PMID: 28860265
35. Organized jointly by the American Thoracic Society, the European Respiratory Society, the European Society of Intensive Care Medicine, and the Société de Réanimation de Langue Française, and approved by ATS Board of Directors, December 2000. International Consensus Conferences in Intensive Care Medicine: noninvasive positive pressure ventilation in acute Respiratory failure. Am J Respir Crit Care Med. 2001 Jan;163(1):283-91. doi: 10.1164/ajrccm.163.1.ats1000. PMID: 11208659.
36. Brindley PG, Beed M, Law JA, Hung O, Levitan R, Murphy MF, Duggan LV. Airway management outside the operating room: how to better prepare. Can J Anaesth. 2017 May;64(5):530-539. English. doi: 10.1007/s12630-017-0834-z. Epub 2017 Feb 6. PMID: 28168630
Schroeder AA. Cricothyroidotomy: when, why, and why not? Am J Otolaryngol. 2000 May-Jun;21(3):195-201. doi: 10.1016/s0196-0709(00)85024-3. PMID: 10834555.
37. Wilson SS, Kwiatkowski GM, Millis SR, Purakal JD, Mahajan AP, Levy PD. Use of nitroglycerin by bolus prevents intensive care unit admission in patients with acute hypertensive heart failure. Am J Emerg Med. 2017 Jan;35(1):126-131. doi: 10.1016/j.ajem.2016.10.038. Epub 2016 Oct 18. PMID: 27825693
38. Wang K, Samai K. Role of high-dose intravenous nitrates in hypertensive acute heart failure. Am J Emerg Med. 2020 Jan;38(1):132-137. doi: 10.1016/j.ajem.2019.06.046. Epub 2019 Jun 25. PMID: 31327485
39. Vital FM, Ladeira MT, Atallah AN. Non-invasive positive pressure ventilation (CPAP or bilevel NPPV) for cardiogenic pulmonary oedema. Cochrane Database Syst Rev. 2013 May 31;(5):CD005351. doi: 10.1002/14651858.CD005351.pub3. Update in: Cochrane Database Syst Rev. 2019 Apr 05;4:CD005351. PMID: 23728654
40. Baeza-Trinidad R, Mosquera-Lozano JD, El Bikri L. Assessment of bendopnea impact on decompensated heart failure. Eur J Heart Fail. 2017 Jan;19(1):111-115. doi: 10.1002/ejhf.610. Epub 2016 Jul 18. PMID: 27427508
41. Wang CS, FitzGerald JM, Schulzer M, Mak E, Ayas NT. Does this dyspneic patient in the emergency department have congestive heart failure? JAMA. 2005 Oct 19;294(15):1944-56. doi: 10.1001/jama.294.15.1944. PMID: 16234501
42. Younis I, Sarwar S, Butt Z, Tanveer S, Qaadir A, Jadoon NA. Clinical characteristics, predictors, and survival among patients with hepatopulmonary syndrome. Ann Hepatol. 2015 May-Jun;14(3):354-60. PMID: 25864216
43. DeVos E, Jacobson L. Approach to Adult Patients with Acute Dyspnea. Emerg Med Clin North Am. 2016 Feb;34(1):129-49. doi: 10.1016/j.emc.2015.08.008. PMID: 26614245; PMCID: PMC7126145
44. Landsberg, J. W. (2018). Chapter two; Manual for Pulmonary and Critical Care Medicine. 398
45. Siemieniuk RAC, Chu DK, Kim LH, Güell-Rous MR, Alhazzani W, Soccal PM, Karanicolas PJ, Farhoumand PD, Siemieniuk JLK, Satia I, Irusen EM, Refaat MM, Mikita JS, Smith M, Cohen DN, Vandvik PO, Agoritsas T, Lytvyn L, Guyatt GH. Oxygen therapy for acutely ill medical patients: a clinical practice guideline. BMJ. 2018 Oct 24;363:k4169. doi: 10.1136/bmj.k4169. PMID: 30355567
Add comment