13 February 2024, by Shahriar Lahouti.
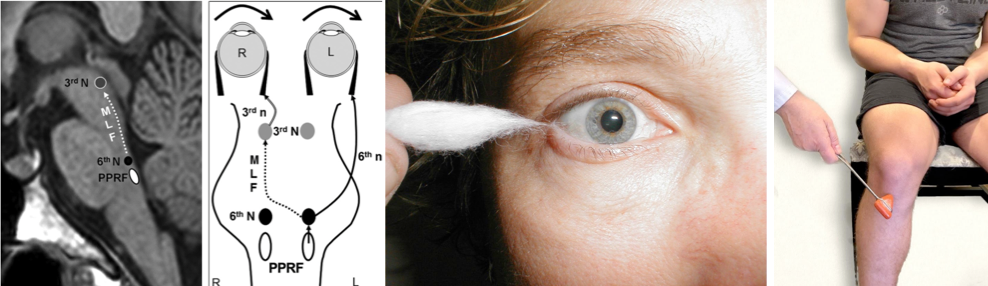
CONTENTS
- Introduction
- Mental status examinatation
- Brainstem and Cranial nerve (CN)
- Motor system
- Somatosensory system
- References
Introduction
The differential diagnosis in neurology is based on two main components, the localization of the neuroanatomic origin(s) of the patient’s symptoms and signs, and the time course over which these symptoms and signs have arisen and evolved. The neurologic examination is a critical tool in localization, confirming or disproving hypotheses generated during the history, or sometimes giving rise to new ones entirely. In this post, a detailed neurologic examination is reviewed concerning the neuroanatomy and the signs and symptoms related to the anatomy under the discussion.
The following table provides a framework for organizing the components of neurologic examination for a comprehensive vs screening neurologic examination.
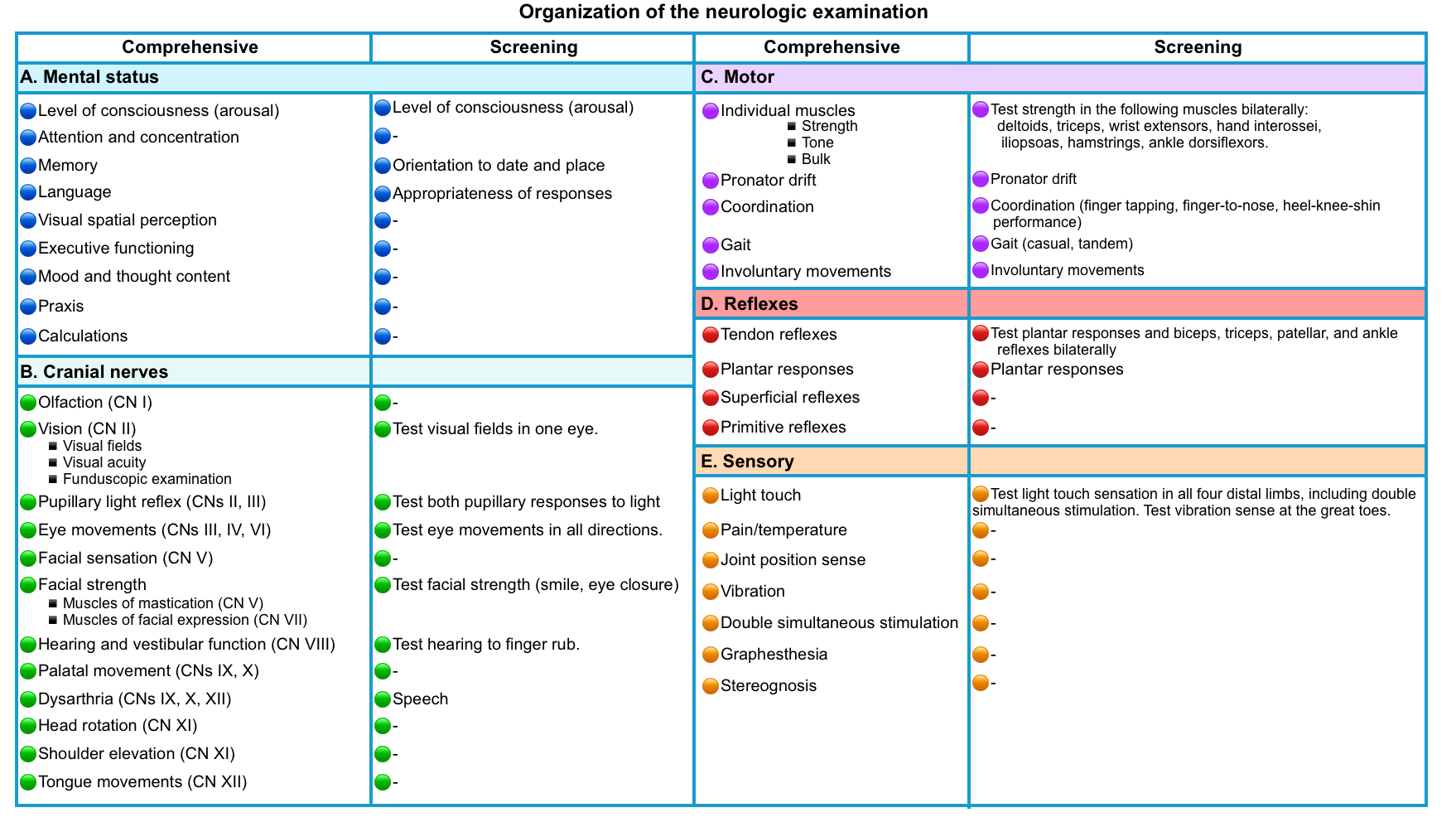
⭐️Quick neurological screening exam: 🎥
⭐️Neurologic exam in coma: 📖
Mental status examination
The examination of the patient’s mental status evaluates two aspects of the mental state:
- The level of consciousness
- The integrity of individual cognitive functions (e.g. attention, memory, language, calculation, abstract reasoning, praxis)
▪️Examination of the level of consciousness: Assessment of the reticular activating system (RAS), thalami, and cerebral hemispheres.
- The level of consciousness refers to the patient’s state of arousal: Is the patient awake? If the patient is awake, is she or he alert? If the patient is alert, is she or he attentive? If the patient is not awake, can she or he be awakened by voice, or is vigorous stimulation required to awaken the patient? Once awakened, is wakefulness maintained or does the patient fall back to sleep?
- An adequate state of arousal is a prerequisite for further mental status testing, which is hierarchical in nature and built upon the assumption of normal levels of arousal. Therefore, results obtained from patients who are sleepy or unresponsive have only limited validity. The neurologic evaluation of a patient in a stupor or coma is discussed here.
▪️Examination of the Integrity of Cognitive Functions: Assessment of the Cerebral Hemispheres.
- Basic
- The neuroanatomic substrates of cognition reside in the cerebral hemispheres.
- If the patient is not awake, or not arousable for long enough to engage in the examination, cognition cannot be tested.
- If the patient is awake and alert, the first cognitive modality to test is attention.
- If the patient’s attention is impaired, the other cognitive domains cannot be effectively evaluated.
- Examination of Attention: A Function of the Frontal and Parietal Lobes
- Attention can be tested by assessing the patient’s ability to recite a string of numbers forward and backward (digit span), asking the patient to recite the days of the week (or the months of the year) backward, asking the patient to spell the word “world” backward (or another word of similar length), or asking the patient to subtract seven serially from 100 (100, 93, 86, and so on).
- Inattention is a core feature of the altered mental state in delirium and inattention to one-half of the world (neglect) can be seen with parietal lesions (most commonly right parietal lesions producing left-sided neglect).
- Examination of Memory: A Function of the Temporal Lobes
- Short-term memory can be tested by asking patients about the recent past (e.g. what they had for breakfast that morning, current events), and long-term memory can be tested by asking about the remote past (e.g. where they were born, and went to school).
- Short-term memory can also be tested by asking the patient to remember three or more words and then asking the patient to recall these words 5 minutes later after the rest of the examination.
- Note that even patients with the most profound deficits in memory due to neurologic conditions should never forget their names. Forgetting one’s name is almost always an indication of a psychiatric condition.
- Memory deficits are a core feature of Alzheimer’s disease and transient global amnesia.
- Short-term memory can be tested by asking patients about the recent past (e.g. what they had for breakfast that morning, current events), and long-term memory can be tested by asking about the remote past (e.g. where they were born, and went to school).
- Examination of Language: A Function of the Frontal and Temporal Lobes (Most Commonly in the Left Hemisphere)
- Language has several components: production (spoken and written), comprehension (hearing and reading), and repetition. The various combinations of deficits in aspects of spoken language are called aphasias.
- Aphasia should be distinguished from dysarthria, which refers to difficulty articulating speech but with preserved language content and structure.
- Common signs of aphasia
- Paraphasias (substitution of one word for a similar word).
- Dysnomia (poor word retrieval).
- Abnormal speech fluency.
- Poor repetition (e.g. inability to repeat a sentence or phrase).
- Naming of objects is almost invariably impaired (regardless of the type of aphasia)
- Types of aphasia
- Broca’s aphasia (nonfluent aphasia, with difficulty producing speech that frustrates the patient): Posterior inferior frontal lobe.
- Wernicke’s aphasia (fluent, with poor comprehension): Superior temporal gyrus.
- Global aphasia is often due to a large lesion near the Sylvian fissure.
- Transcortical aphasias are marked by the ability to repeat normally, but deficiencies in either fluency or comprehension. These localize to areas outside of the perisylvian region.
- Alexia without agraphia (inability to read, yet able to write – aka “word blindness”) *.
- Localizes to the dominant (usually left) medial occipital cortex involving the splenium of the corpus callosum. It is a disconnection syndrome that results from disruption of interhemispheric communication across the corpus callosum. Normally, visual information from the nondominant visual cortex must be transmitted across the corpus callosum to be decoded into language. Writing remains intact because the angular gyrus and associated expressive language centers (Broca area) are intact and connected *.
- Usually due to a PCA (posterior cerebral artery) stroke.
- If detected, this has a strong localizing value *.
- Alexia with agraphia (inability to write or to read): May be associated with lesions in the angular gyrus, or with Wernicke’s aphasia.
- ⚠️ In acute neurological disorders, precise subtyping of aphasia may not localize accurately, as it may take months for an aphasia syndrome to have reliable localizing value.
Brainstem and cranial nerves
Overview of brainstem anatomy
- The three levels of the brainstem from superior to inferior are the midbrain, pons, and medulla.
- The midbrain is just inferior to the bilateral thalami.
- A schematic of brainstem anatomy (left below figure), and location of cranial nerve nuclei (right below figure) are presented here.
▪️Brainstem structures
- As points of orientation for where structures are in the brainstem, the following principles apply at all three levels of the brainstem (left figure above):
- The corticospinal tracts run in the anterior (ventral) aspect of the brainstem.
- The somatosensory pathways for the extremities and torso move a bit over the course of their ascent, but are most often posterior (dorsal) within the brainstem (with the exception of the mid-medulla, where the medial lemnisci are medial and extend anteriorly.
- The cranial nerve nuclei are all posterior (dorsal):
- In general, the motor cranial nerve nuclei are closest to the midline, and their cranial nerves emerge medially/ anteriorly (CN 4 is an exception in that it exits posteriorly).
- The sensory and special sensory cranial nerve nuclei are all more lateral than the motor cranial nerve nuclei: sensory nuclei of CN 5, vestibular and cochlear nuclei (CN 8), and nucleus solitarius (for taste and visceral sensation).
- The ascending neurotransmitter-specific projection pathways are found throughout the brainstem, but the reticular-activating system involved in maintaining arousal and consciousness resides in the upper pons and midbrain.
▪️The cranial nerve nuclei
- The 12 cranial nerves can be divided into three groups of four that mostly correspond to the three brainstem levels with a few exceptions denoted by asterisks:
- Midbrain: 1*-2-3-4
- Pons: 5*-6-7-8*
- Medulla: 9-10-11*-12
- The exceptions are as follows
- CN 1 and CN 11 do not connect with the brainstem.
- CN 5 has nuclei at all three levels of the brainstem (although its fibers enter at the level of the pons)
- For CN 8, the vestibular nuclei are in the medulla and the cochlear nuclei are at the pontomedullary junction.
- CN 2 can be considered a partial exception: Although CN 2’s main projections are the visual pathways, which do not project to the brainstem, the afferent limb of the pupillary light reflex is communicated by CN 2 to the midbrain.
Optic nerve: CN2
- The optic nerve is formed by the axons of the retinal ganglion cells. The axons acquire myelin sheaths as they leave the optic disc.
- CN2 is not a true peripheral nerve.
- A true peripheral nerve whether cranial or spinal, contains Schwann cells and collagenous sheaths and is capable of regeneration.
- The optic nerve contains neuroglial cells of central type (astrocytes and oligodendrocytes) and is not capable of regeneration in mammals.
- In addition, the nerve is invested with meninges containing an extension of the subarachnoid space, a feature largely responsible for the changed appearance of the fundus oculi when the intracranial pressure is high.
- CN2 is not a true peripheral nerve.
- At the optic chiasm, fibers from the nasal hemiretina (medial half of the retina) enter the contralateral optic tract, whereas those from the temporal (lateral) hemiretina remain uncrossed and enter the ipsilateral tract.
- Each optic tract winds around the midbrain and divides into a medial and a lateral root.
- The medial root of the optic tract contains 10% of the optic nerve fibers. It enters the side of the midbrain and contains four distinct sets of fibers:
- Some fibers enter the superior colliculus and provide for automatic scanning, such as reading this page.
- Some fibers are relayed from the superior colliculus to the pulvinar of the thalamus; they belong to the extra-geniculate pathway to the
visual association cortex. - Some fibers enter the pretectal nucleus and serve the pupillary light reflex.
- Some fibers enter the parvocellular reticular formation, where they have an arousal function.
- The lateral root of the optic tract terminates in the lateral geniculate body (LGB) of the thalamus.
- The medial root of the optic tract contains 10% of the optic nerve fibers. It enters the side of the midbrain and contains four distinct sets of fibers:
- The optic radiation (geniculocalcarine tract) travels from the lateral geniculate body to the primary visual cortex.
- Meyer loop: Fibers (inferior retial fibers, carrying superior visual field information) sweep forward into the temporal lobe. These fibers end up in the lower half of the primary visual cortex (lingual gyrus).
- The primary visual cortex (PVC) occupies the walls of the calcarine sulcus.
- It emerges onto the medial surface of the hemisphere for 5 mm both above and below the sulcus, and onto the occipital pole of the brain for 10 mm.
- The lingual gyrus and the cuneus are the gyri immediately inferior and superior, respectively, to the calcarine sulcus.
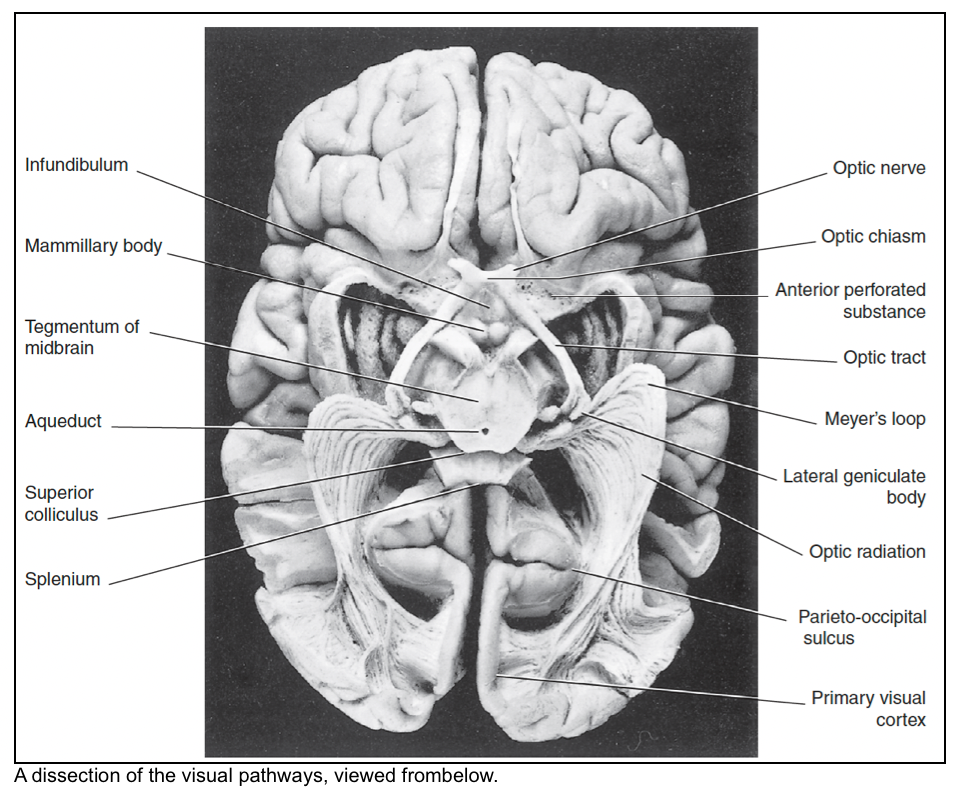
Visual field
▪️Examination
- There are many technique for testing visual field. 🎥
- Regardless of the technique used, each eye must be tested separately.
▪️Definitions of visual field defects
- Scotoma: A scotoma is an area of partial alteration in the field of vision consisting of a partially diminished or entirely degenerated visual acuity that is surrounded by a field of normal or relatively well-preserved
- Hemianopia is a loss of vision in half the visual field, usually on one side of the vertical midline.
- Homonymous hemianopia is the loss of half of the visual field on the same side in both eyes.
- Heteronymous hemianopsia is the loss of half of the visual field on different sides in both eyes. It is separated into two categories:
- Bilateral hemianopsia refers to the loss of the fields surrounding the nose.
- Bitemporal hemianopsia refers to the loss of the fields closest to the temples.
- Quadrantanopia (quadrantanopsia or quadrantic hemianopsia) is decreased vision or blindness in one-quarter of the visual field.
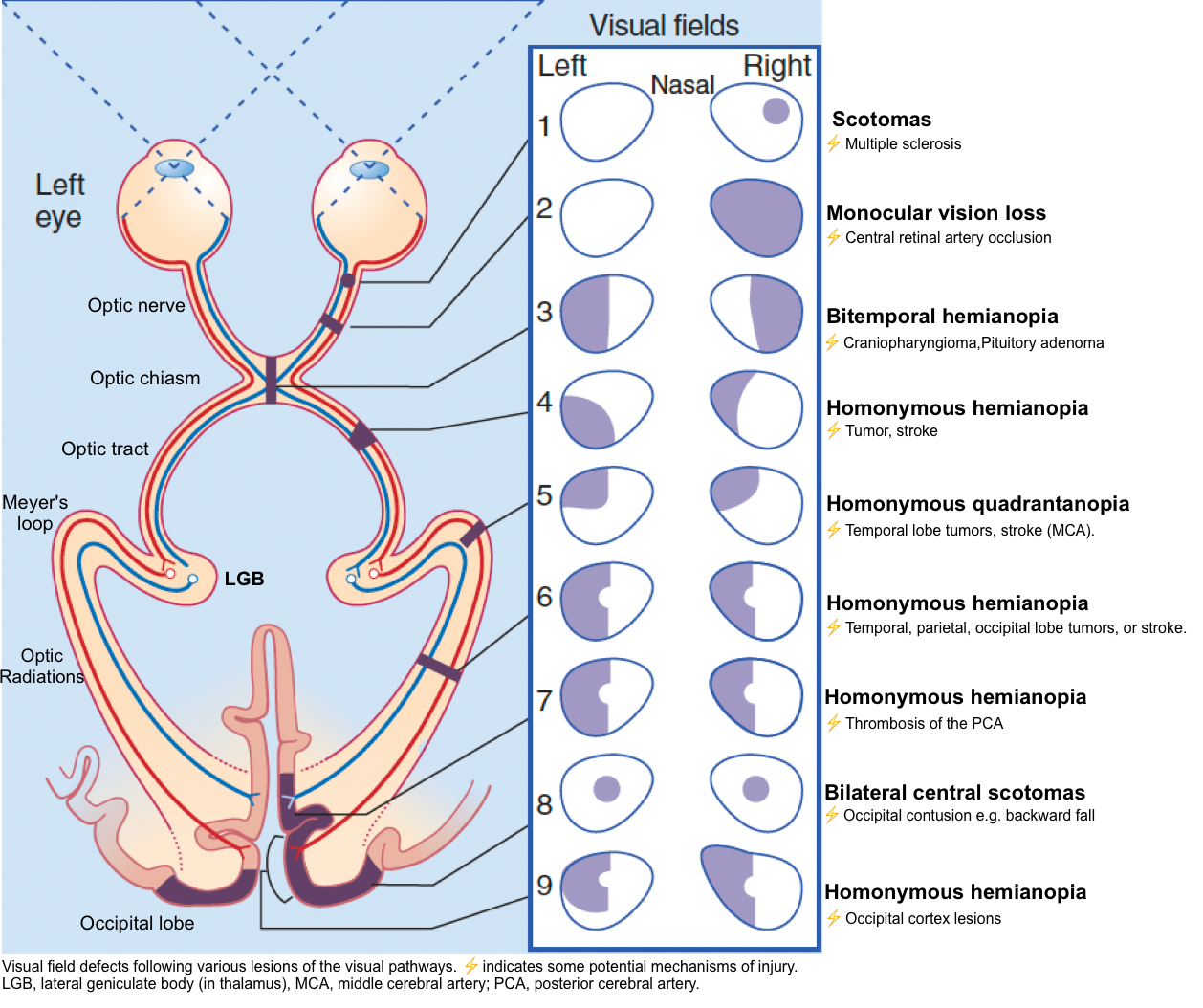
🔴Monocular vision loss
- Pathology before the optic chiasm:
- Ocular problem:
- Lens (e.g. cataract).
- Anterior chamber (e.g. uveitis).
- Retina (e.g. retinal ischemia).
- Optic nerve problem (e.g. optic neuropathy).
- Ocular problem:
- Lesion anterior to optic chiasma causes ipsilateral monocular vision loss. Pupil light reflex (PLR) is affected.
🔴Bitemporal hemianopsia
- Pathology at the optic chiasm.
- Causes:
- Pituitary lesions (most common cause).
- Sellar meningioma or craniopharyngioma.
- Aneurysm of the distal carotid artery.
🔴Homonymous hemianopia
- One study involving 904 patients found the following lesions among patients with homonymous hemianopsia *:
- Occipital lobe (45%).
- Optic radiations (32%).
- Optic tracts (10%).
- Lateral geniculate body of the thalamus (1%).
🔴Homonymous quadrantanopia
- Generally localizes to the optic radiation or the occipital lobe.
🔴Cortical blindness
- Severe, bilateral occipital lobe damage may cause the brain to be unable to understand visual information. This can be caused by bilateral PCA (posterior cerebral artery) strokes.
- Anton syndrome: The patient is unaware that they are blind.
- Charles Bonnet syndrome: Release hallucinations occur, often involving small people. The patient generally knows that these are not real.
Pupil light response: CN 2,3
Neuroanatomy
▪️Pupillary constriction: The parasympathetic pathway 🎥
- Constriction of the pupils in response to light optimizes visual acuity and protects the retina from overexposure to bright light. It involves four sets of neurons (left figure below):
- The afferent limb commences in melanopsin-containing retinal ganglion cells, with inputs from photoreceptors (rods and cones), and travels within the optic nerve.
- Fibers leaving the chiasm enter both optic tracts and terminate in the pretectal nuclei, situated just rostral to the superior colliculus on each side.
- Each pretectal nucleus is linked by interneurons to both Edinger–Westphal (parasympathetic) nuclei; the contralateral nucleus is reached by way of the posterior commissure (PC).
- Preganglionic parasympathetic fibers enter the oculomotor nerve, leave the branch to the inferior oblique, and synapse in the ciliary ganglion.
- Postganglionic fibers run in the short ciliary nerves and enter the iris to supply the sphincter (constrictor) pupillae.
- 👉The normal response to light is consensual; that is, both pupils constrict when the light is applied to one eye only.
▪️Sympathetic pathway to the eyes (right figure below)
- Central fibers descending from the hypothalamus cross to the other side of the midbrain. In the pons and medulla, they are joined by ipsilateral fibers descending from the reticular formation.
- Preganglionic fibers emerge in the first thoracic ventral nerve root and run up in the sympathetic chain to the superior cervical ganglion.
- Postganglionic fibers from the superior cervical ganglion run along the external and internal carotid arteries and their branches.
- Interruption of this ‘three-neuron’ oculosympathetic pathway anywhere along its course can result in Horner syndrome (small pupil, ipsilateral ptosis, and variable anhidrosis depending on the site of the lesion).
- The external carotid sympathetic fibers accompany all of the branches of the external carotid artery. Those accompanying the facial artery supply the arterioles of the cheek and lips and are particularly responsive to emotional states. Those accompanying the maxillary artery supply the cavernous tissue covering the nasal conchae
(turbinate bones). - Two sets of sympathetic fibers accompany the internal carotid artery.
- One set leaves it to join the ophthalmic division of the CN-5 in the cavernous sinus, then leaves this in the long and short ciliary nerves to supply the vessels and smooth muscles of the eyeball (ptosis results from paralysis of the smooth muscle fibers within the aponeurosis of the levator palpebrae superioris, the superior tarsal muscle of Muller).
- The second set forms a plexus around the internal carotid artery and its branches, including the ophthalmic artery. The ophthalmic artery gives off supratrochlear and supraorbital branches, which carry sympathetic fibers to the skin of the forehead and scalp.
- Interruption of the postganglionic fibers at the jugular foramen, or in the cavernous sinus, produces anhidrosis (loss of sweating) on the forehead and scalp.
Pupil exam
When performing a pupillary exam, it sometimes helps to illuminate pupils indirectly from the side, so you can see what is happening. 🎥
- Observe the pupil size and shape at rest, looking for anisocoria (one pupil larger than the other)
- Observe the direct response (constriction of the illuminated pupil)
- Observe the consensual response (constriction of the opposite pupil)
- Repeat with the opposite pupil
- Check for accommodation (constriction of the pupil when viewing a close object)
Abnormal pupil size or reactivity
- Unilateral abnormal pupil size or reactivity (Anisocoria)
- Bilateral abnormal pupil size or reactivity
Approach to anisocoria
- History: Ocular trauma or surgery? old photographs (chronic anisocoria? chronic ptosis?). Exposure to topical medications? (including nebulized ipratropium).
- Determine if it is a sympathetic lesion or a parasympathetic lesion
- Parasympathetic lesion (e.g. CN3-oculomotor palsy):
- Anisocoria is greater in the light (inability to constrict).
- The larger pupil has a sluggish response to light (impaired constriction).
- The larger pupil is abnormal (unilateral mydriasis).
- (If anisocoria is similar in light or dark this suggests the possibility of physiological anisocoria).
- Sympathetic lesion (e.g. Horner’s syndrome):
- Anisocoria is greater in the dark (inability to dilate). This is especially noticeable within ~5-10 seconds of turning down the lights (dilation lag).
- Both eyes are briskly responsive to light (able to constrict).
- The small pupil is abnormal (unilateral miosis).
- This often implies the presence of Horner’s syndrome (especially if there is also ptosis).
- Parasympathetic lesion (e.g. CN3-oculomotor palsy):
Unilateral pupil size or reactivity abnormality (anisocoria) |
🔴Physiologic anisocoria
- The degree of anisocoria is <1 mm.
- Normal response to light and accommodation (this exonerates CN3).
- Lack of ptosis.
- The degree of anisocoria is similar in light and darkness (this exonerates the sympathetic system).
- Chronic (may be seen on prior photographs, such as a driver’s license).
🔴RAPD (relative afferent pupillary defect, aka Marcus Gunn pupil) 🎥
- Usually caused by optic nerve pathology (or profound, extensive retinal disease).
- One pupil is less responsive to light but does constrict when light is shone on the contralateral pupil (a consensual response).
- Swinging flashlight test: A flashlight is swung back and forth between the eyes, illuminating each eye for about two seconds. To avoid a near pupil response, the patient should fixate on a distant object and light should be shown on the eyes from below. The test is positive if the affected eye has reduced constriction and more rapid dilation (escape). In more severe cases, the affected eye may frankly dilate when light is shining on it.
🔴Oval pupil
- This may indicate increased intracranial pressure (e.g., due to an acute hemispheric mass effect). Ovoid pupils commonly precede anisocoria (unequal pupil size).
- Oval or irregular pupils may be associated with midbrain pathology.
🔴Unilateral parasympathetic lesion (mydriasis)
- CN3-oculomotor dysfunction
- Midbrain lesion affecting the CN3-oculomotor nucleus. This is especially suggested if the involved eye is turned downwards and outwards (although external compression can affect pupil dilation before affecting eye movement). The dilated pupil may be sluggish, or entirely fixed/dilated. Causes include:
- Herniation syndrome compressing the nerve sheath.
- Midbrain lesion affecting the CN3-oculomotor nucleus.
- Midbrain lesion affecting the CN3-oculomotor nucleus. This is especially suggested if the involved eye is turned downwards and outwards (although external compression can affect pupil dilation before affecting eye movement). The dilated pupil may be sluggish, or entirely fixed/dilated. Causes include:
- Focal seizure
- Iatrogenic anticholinergic medication:
- Adie’s tonic pupil: Isolated pupil dilation with light-near dissociation (reaction to accommodation is normal). 🎥
🔴Unilateral sympathetic lesion, “miosis” (Horner’s syndrome)
Bilateral pupil size or reactivity abnormality |
🔴Bilaterally dilated and fixed pupils
- Bilateral midbrain damage causing loss of parasympathetic output from CN3-oculomotor (most often due to early transtentorial herniation).
- Extremely deep metabolic coma (e.g. hypothermia, barbiturates, bupropion, lidocaine, sympathomimetics).
- Anticholinergic drugs at high doses (especially atropine).
- Botulism.
🔴Bilaterally dilated and reactive pupils
- Anticholinergic drugs.
- Withdrawal from alcohol or benzodiazepines.
- Sympathomimetics (e.g. amphetamine, cocaine, methamphetamines, MDMA).
- Serotonin syndrome.
- Generalized seizure, including the postictal period.
🔴Bilateral mid-position and fixed
- Bilateral midbrain damage with loss of sympathetic and parasympathetic output (most commonly seen after herniation, or in patients with brain death).
- Profound barbiturate coma (but not high doses of IV benzodiazepines or propofol).
- Hypothermia.
🔴Bilateral mid-position and reactive
- Normal pupils exclude a large lesion at the level of the midbrain. For a comatose patient, the remaining possibilities include:
- Most likely, this may be a toxic/metabolic coma, causing diffuse dysfunction of the cerebral cortex.
- Lesions in the dorsolateral, upper-mid pons.
- Bilateral thalamic dysfunction.
- Neuromuscular blocking medications (note that smooth muscles of the iris don’t get paralyzed).
🔴Bilateral small pupils
- Medications (e.g. cholinergic agonists, opioids, clonidine, ACE inhibitors).
- Bilateral central Horner’s syndrome
- Usually due to extensive pontine hemorrhage (“pontine pupils”).
- May occur in the early phase of central herniation syndromes (either upwards or downwards herniation).
- Argyll Robertson pupils (bilateral small, irregular, and often asymmetric pupils that retain responsiveness to accommodation, but not to light. 🎥
Horner’s syndrome
- The classic features of Horner’s syndrome are miosis, ptosis, and anhidrosis (small pupil, drooping eyelid, and lack of sweat). In critical care practice it’s generally impossible to accurately determine the presence of anhidrosis, so this triad can be boiled down to the dyad of miosis and ptosis.
- Ptosis involves the upper and lower lids. Thus, the most accurate way to evaluate this is the distance between the upper and lower eyelids (“palpebral length”).
- Examination in a darkened room may cause the normal pupil to dilate, thereby accentuating the difference between the pupils. The difference is greatest shortly after darkening the room (dilation lag; see the video below).
- Both pupils are briskly reactive to light (unlike anisocoria due to parasympathetic dysfunction).
- Examples:
▪️Causes of Horner’s syndrome
- First-order nerves begin in the hypothalamus, travel through the lateral brainstem, and end in the intermediolateral column of the spinal cord (ciliospinal center of Budge).
- Large thalamic hemorrhage, or posterolateral thalamic lesion. 📖
- Early phase of central herniation syndromes (either upwards or downwards herniation).
- Lateral brainstem lesion:
- Inferior lateral pons (Marie-Foix syndrome ).
- Lateral medulla (Wallenberg’s syndrome ).
- Spinal cord lesion (C1-T2 level).
- Second-order nerves travel from the spinal cord over the lung apex, to the superior cervical ganglion.
- Brachial plexus lesion.
- Cancer in the lung apex (Pancoast tumor).
- Thoracic surgery or chest tube placement.
- Third-order neurons travel from the superior cervical ganglion to the eye (running along the internal carotid artery and then through the cavernous sinus).
- Internal carotid artery dissection.
- Neck surgery.
- Cavernous sinus pathology.
Extraocular movements: CN 3,4,6
Examining eye movement
- Check extraocular movements (eye movements) by having the patient look in all directions without moving their head. While doing this, ask them if they experience any double vision. 🎥
- Test smooth pursuit by having the patient follow an object moved across their full range of horizontal and vertical eye movements.
- Test convergence movements by having the patient fixate on an object as it is moved slowly toward a point right between the patient’s eyes.
- Also, observe the eyes at rest to see if there are any abnormalities such as spontaneous nystagmus (which we discuss shortly) or dysconjugate gaze (eyes not both fixated on the same point), resulting in diplopia (double vision).
Oculomotor nerve: CN 3
▪️Neuroanatomy
- Origin
- The nucleus of the third nerve is at the level of the superior colliculus. It is partly embedded in the periaqueductal grey matter.
- The nucleus is composed of
- Five individual subnuclei for the supply of striated muscles (ipsilateral subnuclei innervate the inferior rectus, inferior oblique, and medial rectus, but the contralateral superior rectus muscle; the levator palpebrae superioris is innervated by a single midline nucleus)
- One parasympathetic nucleus (Edinger–Westphal nucleus).
- Pathway
- The nerve passes through the tegmentum of the midbrain and emerges into the interpeduncular fossa. It crosses the apex of the petrous temporal bone, pierces the dural roof of the cavernous sinus, runs in the lateral wall of the sinus, and breaks into upper and lower divisions within the superior orbital fissure.
- The upper division supplies the superior rectus and the levator palpebrae superiors.
- The lower division supplies the inferior and medial recti and the inferior oblique.
- The parasympathetic fibers originate in the Edinger–Westphal nucleus.
- They accompany the main nerve as far as the orbit, then leave the branch to the inferior oblique and synapse in the ciliary ganglion.
- Postganglionic fibers emerge from the ganglion in the short ciliary nerves, which pierce the lamina cribrosa of the sclera and supply the ciliary and sphincter pupillary muscles.
- The nerve passes through the tegmentum of the midbrain and emerges into the interpeduncular fossa. It crosses the apex of the petrous temporal bone, pierces the dural roof of the cavernous sinus, runs in the lateral wall of the sinus, and breaks into upper and lower divisions within the superior orbital fissure.
▪️Features of CN3 palsy
- Pupil dilation. This may be the first finding due to external compression of the nerve since parasympathetic fibers run along the outside of the nerve.
- Ptosis (CN3 supplies the levator palpebrae, which elevates the eyelid).
- Eye position is down and out (due to unopposed actions of abducens and superior oblique muscles).
▪️Localizing the lesion
- Localization to the brainstem may be suggested by:
- Bilateral ptosis and bilateral superior rectus palsy (suggests a lesion of the CN3 nucleus, given bilateral innervation of these muscles).
- Any sign of crossed long-tract findings, for example:
- Contralateral hemiplegia.
- Contralateral ataxia.
- Contralateral tremor.
- Pupil-sparing CN3-oculomotor palsy
- Intrinsic nerve micro-infarction due to diabetes/hypertension may cause abnormal eye position without affecting the pupils because this affects the innermost fibers of the nerve. This clinical presentation is less worrisome for a mass lesion.
- However, another possibility that should be considered here is superior divisional CN3 palsy, which presents as ptosis and limited upward eye movement without pupillary involvement. This is most commonly associated with lesions in the anterior cavernous sinus or superior orbital fissure that compress the superior division of the nerve. However, other causes may include intrinsic brainstem disease, aneurysms, or leptomeningeal carcinomatosis.
▪️Important causes of CN3 lesions
- Brainstem lesions involving the CN3 nucleus.
- Damage to the nerve as it passes through the subarachnoid space:
- Uncal herniation (usually ipsilateral).
- Meningitis.
- Aneurysm of the posterior communicating artery, posterior cerebral artery, superior cerebellar artery, or internal carotid artery. Aneurysms of the anterior communicating artery or posterior cerebral artery may rarely cause contralateral CN3 dysfunction, possibly due to compression of the nerve against the tentorium.
- Skull base tumors.
- Cavernous sinus disorder (additional nerves are usually involved including trochlear, abducens, and division V1 of the trigeminal).
- Other causes: Trauma, postoperative, idiopathic intracranial hypertension.
Trochlear nerve: CN4
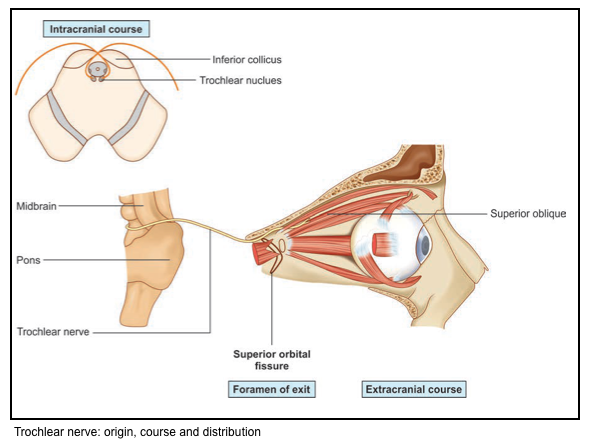
▪️Origin & pathway
- The nucleus of the fourth nerve is at the level of the inferior colliculus. The nerve itself is unique in two respects
- it is the only nerve to emerge from the dorsum of the brainstem and the only nerve to fully decussate.
- The IV nerve winds around the crus of the midbrain and travels through the cavernous sinus in company with the III nerve.
- It passes through the superior orbital fissure and supplies the superior oblique muscle.
Abducens nerve: CN 6
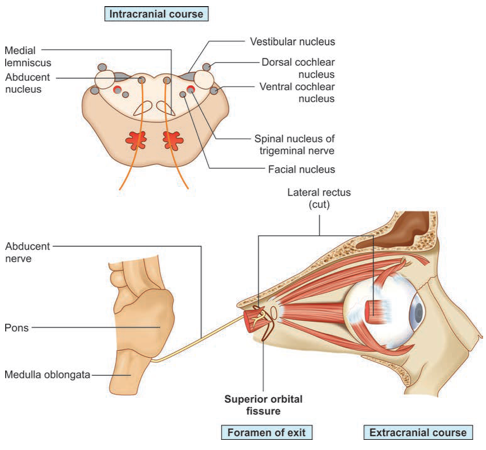
▪️Neuroanatomy
- Origin
- The nucleus of the sixth nerve (in the floor of the fourth ventricle), is at the level of the facial colliculus in the lower pons.
- Pathway
- The nerve descends to emerge at the lower border of the pons and runs up the pontine subarachnoid cistern beside the basilar artery.
- It angles over the apex of the petrous temporal bone and passes through the cavernous sinus beside the internal carotid artery.
- It enters the orbit through the superior orbital fissure and supplies the lateral rectus muscle, which abducts the eye.
▪️Clinical manifestation of CN6 palsy: Inability to abduct the ipsilateral eye (i.e. temporal, or outward movement).
▪️Localizing the lesion of CN6 palsy
- Abducens nucleus lesion in the pons may be suggested by any of the following additional features:
- Involvement of nearby CN7-facial, causing facial weakness.
- Inability to adduct the contralateral eye (complete lateral gaze palsy, due to damage to the pontine lateral gaze center).
- Contralateral hemiparesis. If the pontine lesion also involves the corticospinal tract, eyes deviate away from the lesion (and toward the paretic limb). This is sometimes called “wrong way eyes,” since it is the opposite of the more commonly seen pattern that occurs with destructive lesions of the frontal lobe (wherein eyes look away from the paretic limb). Wrong-way eyes usually indicate a contralateral pontine lesion involving the abducens nucleus, but this can also be caused by thalamic hemorrhage.(Berkowitz 2017)
- Abducens nucleus damage is a component of the inferior medial pontine syndrome (Foville syndrome)
▪️Causes of CN6 palsy
- Increased intracranial pressure:
- Abducens nerve palsy is frequently a nonspecific sign of increased intracranial pressure. (This may result from any process that exerts downward pressure on the brainstem.)
- Elevated intracranial pressure may especially be suggested by bilateral CN6 palsies.
- Pontine pathology (additional abnormalities would usually be expected, as described above).
- Wernicke encephalopathy.
- Cavernous sinus lesion (should see other cranial nerves involved as well, especially CN3-oculomotor and/or CN4-trochlear).
- Meningitis, syphilis, or Lyme disease.
- Skull base neoplasm.
- Microvascular ischemia (e.g. diabetes, hypertension, smoking).
- Trauma.
- Multiple sclerosis.
Control of eye movements
▪️Functional terms
- The eyes normally move as a pair. This conjugate movement is of two fundamentally different kinds, as follows:
- Gaze shifting. Cortical and subcortical areas are responsible for these volitional eye movements.
- Saccadic, or conjugate high-velocity eye movements that redirect fixation to a new object of interest and onto the fovea of the eye.
- Vergence, or dysconjugate eye movements that shift gaze from far to near targets (e.g. fixation reflex).
- Gaze holding. The visual system (retina to visual cortex) provides feedback concerning the position of the target of gaze and the eye position that keeps the target on the retina when the target or we move.
- Tracking, or smooth pursuit, occurs as the eyes follow an object of interest that is moving slowly across the visual field; eye velocity matches that of the visual target and maintains visual acuity by keeping the image on the fovea.
- Vestibuloocular reflex: gaze can be held on an object of interest during movements of the head and is dependent upon displacement of endolymph in the kinetic labyrinth.
- Gaze shifting. Cortical and subcortical areas are responsible for these volitional eye movements.
Neuroanatomy of gaze
⭐️The neuroanatomy of horizontal and vertical gaze is illustrated below. Watch this video 🎥
▪️Horizontal conjugate gaze
- The paired centers (left and right) for horizontal eye movements are in the paramedian pontine reticular formation (PPRF), which are located in the pons.
- When each is activated it will result in a conjugate (both eyes) movement to its own side; for example, activation of the left PPRF moves both eyes so that they gaze to the left. How does it work?
- The PPRF on each side is connected with the ipsilateral cranial nucleus 6.
- Each cranial nucleus 6 is connected with the contralateral cranial nucleus 3 through the medial longitudinal fasciculus (MLF).
- The MLF crosses from the CN 6 nucleus en route to the contralateral CN 3 nucleus almost immediately, spending most of its course contralateral to its point of origin. For this reason, the MLF is named for the side of the CN 3 nucleus with which it connects rather than the CN 6 nucleus from which it originates: The left MLF travels from the right CN 6 nucleus to the left CN 3 nucleus, and the right MLF travels from the left CN 6 nucleus to the right CN 3 nucleus.
- When each is activated it will result in a conjugate (both eyes) movement to its own side; for example, activation of the left PPRF moves both eyes so that they gaze to the left. How does it work?
- Voluntary horizontal gaze
- The signal to voluntarily move the eyes comes from the frontal eye fields. The frontal eye fields communicate directly with the contralateral horizontal (PPRF) and vertical gaze centers.
- The flow of information for voluntary horizontal gaze is from frontal eye fields → contralateral PPRF → CN 6 nucleus → contralateral CN 3 nucleus (via MLF). For example, to voluntarily look to the left; the right frontal eye field sends signals to the left PPRF. The left PPRF signals the left CN 6 nucleus to activate the left lateral rectus. The left CN 6 nucleus simultaneously communicates to the contralateral (right) CN 3 nucleus by way of the right MLF to signal the right CN 3 to activate the right medial rectus.
- The signal to voluntarily move the eyes comes from the frontal eye fields. The frontal eye fields communicate directly with the contralateral horizontal (PPRF) and vertical gaze centers.
- Reflexive horizontal gaze i.e. vestibulo-ocular reflex (right figure below).
- Each vestibular nucleus (in the dorsolateral medulla) communicates with cranial nerve nuclei for eye movements (CNs 3, 4, 6) via the medial longitudinal fasciculus (MLF).
- Vestibular information is also communicated to the vestibulocerebellum (the flocculus/nodulus of the cerebellum) via the inferior cerebellar peduncles.
- Oculocephalic reflex
- When the head is rotated, the eyes normally turn in the opposite direction (thereby staying fixated in roughly the same direction).
- Turning the head toward the left causes the left vestibular system to become more activated than the right side, and this activation pattern is communicated through the MLF to the right abducens nucleus to send the eyes to the right (via the right CN 6 and left CN 3).
- ⭐️Keep in mind that in awake patients, the oculocephalic reflex is overcome by voluntary gaze control.
- When the head is rotated, the eyes normally turn in the opposite direction (thereby staying fixated in roughly the same direction).
- Cold Caloric Testing
- This involves slowly flushing the ear with 50 ml of ice water, with the head of the bed inclined at a 30-degree angle (to properly align the semicircular canal). At least five minutes should be allowed to pass in between testing each ear.
- Cold water “turns off” the vestibular apparatus. If one side is turned off, the other side’s normal baseline activity is “greater than off,” so the brain thinks the head is moving toward the not-cold side. This causes the eyes to move away from the not-cold side and toward the cold side.
- If the frontal lobes are intact, there will be nystagmus with the fast phase in the direction opposite the slow phase (i.e. the fast phase of the nystagmus is away from the cold side).
- Each vestibular nucleus (in the dorsolateral medulla) communicates with cranial nerve nuclei for eye movements (CNs 3, 4, 6) via the medial longitudinal fasciculus (MLF).
▪️Vertical conjugate gaze
- Vertical eye movements are controlled by two brainstem nuclei, the rostral interstitial nucleus of the MLF (vertical and torsional saccades) and the interstitial nucleus of Cajal (vertical gaze holding; eye-head coordination), both located in the dorsal midbrain close to the CN 3 and CN 4 nuclei, as would be expected (CN 6 does not participate in vertical gaze).
- Like the brainstem horizontal gaze centers, these nuclei are under the control of the cortical eye fields.
- Lesions of the dorsal midbrain or in the region of the fourth ventricle (e.g. pineal pathology, tectal glioma, hydrocephalus with expansion of the 4th ventricle) and neurodegenerative diseases) will cause impaired conjugate vertical gaze.
- Parinaud’s syndrome
- Clinical features
- Impaired upgaze with downgaze preference.
- Light-near dissociation of pupillary reactions (constriction on accommodation but not in response to light).
- Eyelid retraction (called Collier’s sign), causing a wide-eyed appearance to the eyes.
- Convergence-retraction nystagmus, in which the eyes are pulled medially and retracted inward. This can be brought out in an upward gaze. 🎥
- Parinaud’s syndrome is caused by pathologies that compress the dorsal midbrain around the cerebral aqueduct and involve the corpora quadrigemina and posterior commissure *. Common causes include:
- Hydrocephalus, particularly fourth ventricular outflow obstruction.
- Tumor (e.g. pineal tumor or tectal glioma).
- Multiple sclerosis.
- Stroke.
- Clinical features
- Parinaud’s syndrome
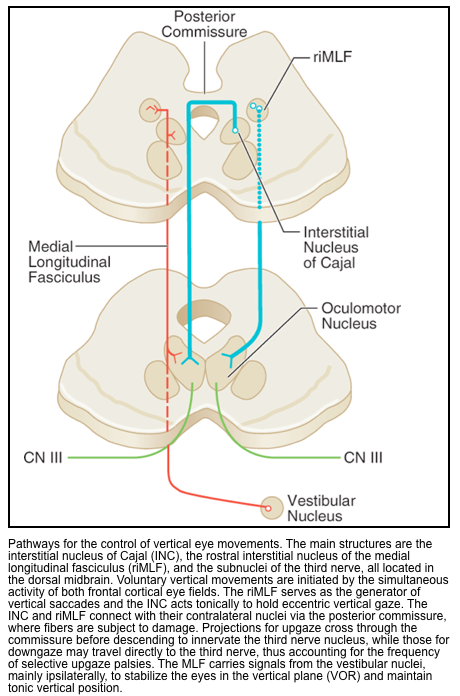
Gaze palsy
An eye movement abnormality in which the two eyes move conjugately but have limited movement in one direction is called a gaze palsy. It is due to malfunction of one of the “gaze centers” (cortical and brainstem regions responsible for conjugate gaze) or to interruption of the pathways leading from them.
- When the lesion is in a cortical gaze center, only voluntary gaze is impaired; reflexes can still activate the brainstem neurons responsible for gaze. This is called a supranuclear gaze palsy.
- When the lesion is in a brainstem gaze center, the neurons there cannot be activated either voluntarily or by reflex (such as the oculocephalic reflex or “doll’s eyes response”). This is called nuclear gaze palsy.
Examination: How to test gaze abnormalities?
▪️Awake & communicative patient
- Ask the patient to follow your finger, or ask them to look to the right and the left. This stimulates a pathway involving the frontal eye fields and the PPRF (paramedian pontine reticular formation).
- It also requires the patient to have intact hearing and language comprehension.
▪️Comatose patients
- In the comatose patient, presence of bilateral conjugate roving eye movements that appear full, indicate an intact brainstem and further reflex testing is not required.
- In the absence of this finding, horizontal eye movements can be tested with the two VORs (discussed below):
- Oculocephalic reflex (doll’s eye)
- Principles
- This is easily tested, as a front-line evaluation of eye movements in comatose patients.
- Presence of oculocephalic reflex argues against a structural cause of coma (i.e. brainstem damage).However, this reflex may be absent in deep metabolic coma.
- ⭐️Therefore, absence of symmetric reflexive eye movements doesn’t necessarily indicate a brainstem injury.
- Presence of oculocephalic reflex may suggest:
- Comatose state with intact brainstem.
- Comatose patients with toxic-metabolic encephalopathy.
- Absence of oculocephalic reflex can be seen in:
- Coma with injured brainstem (structural coma).
- Psychogenic coma (awake patients).
- Deep metabolic coma
- ⭐️If it’s unclear whether an abnormal oculocephalic reflex is due to voluntary gaze control versus brainstem pathology, this may also be sorted out using cold caloric testing.
- Principles
- Cold caloric testing (vestibulo-ocular reflex)
- In a comatose patient with intact brainstem reflexes, this will cause the eyes to rotate toward the stimulated ear.
- ⚠️ Cold calorics may elicit nausea and vomiting in awake or mildly somnolent patients.
- Response to cold calorics often reveals an objective assessment of the patient’s level of consciousness, which is especially useful in diagnosis of psychogenic coma.
- Responses may be classified along a spectrum, as follows:
- Completely normal response
- Contralateral nystagmus occurs, without significant eye deviation.
- The cerebrum maintains complete control of eye position, albeit with some slow deviations (due to cold caloric stimulation) that are promptly corrected via nystagmus (fast-phase nystagmus occurs away from the cold ear).
- In a patient who appears to be clinically comatose, this unexpected finding would suggest a functional coma (pseudocoma), malingering, or akinetic mutism.
- Contralateral nystagmus occurs, without significant eye deviation.
- Mildly abnormal response
- Eyes deviate towards the cold ear, with contralateral nystagmus.
- The cerebrum loses control of the eye position, but it is still attempting to drag the eye back to a normal position.
- This could be seen with bilateral hemispheric dysfunction causing lethargy or obtundation.
- Eyes deviate towards the cold ear, with contralateral nystagmus.
- Ipsilateral eye deviation only
- Eyes deviate towards the cold ear, without nystagmus.
- This reflects the intact function of the brainstem, with no input from the cerebrum.
- Clinically this pattern may be seen in a patient with bilateral hemispheric dysfunction causing stupor/coma.
- Eyes deviate towards the cold ear, without nystagmus.
- No response at all
- Absent vestibulo-ocular reflexes usually indicate brainstem pathology. However, this may also be seen in very deep toxic/metabolic coma (e.g. phenytoin, tricyclic antidepressants, sedatives) or following the administration of paralytics. Thus, the differential diagnosis here may include various brain-death mimics.
- Completely normal response
Horizontal gaze abnormalities
🔴Frontal lobe damage causes a gaze preference.
- The direction of preference:
- Cortical damage (e.g., stroke): Eyes deviate towards the lesion & away from the paretic limb.
- Focal seizure: Eyes deviate away from the lesion & towards the seizing limb.
- Unilateral pontine stroke affecting the not-yet-crossed corticospinal tract and the lateral gaze center on one side will be unable to look toward the side of the lesion, which can produce gaze deviation toward the side of the hemiparesis, which is away from the side of the lesion.
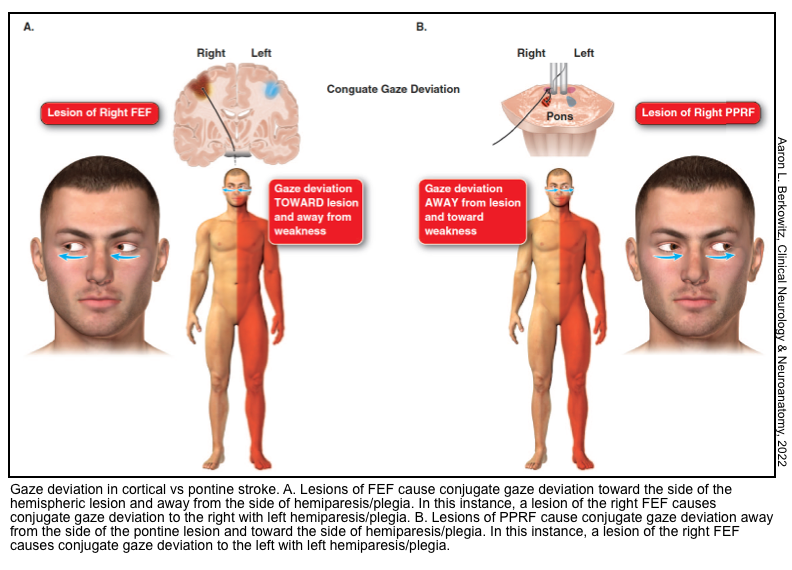
🔴Peripheral lesion of CN6-abducens
-
This causes an ipsilateral inability to abduct the eye (in response to any stimuli). A schematic diagram of the left CN6 is shown below.
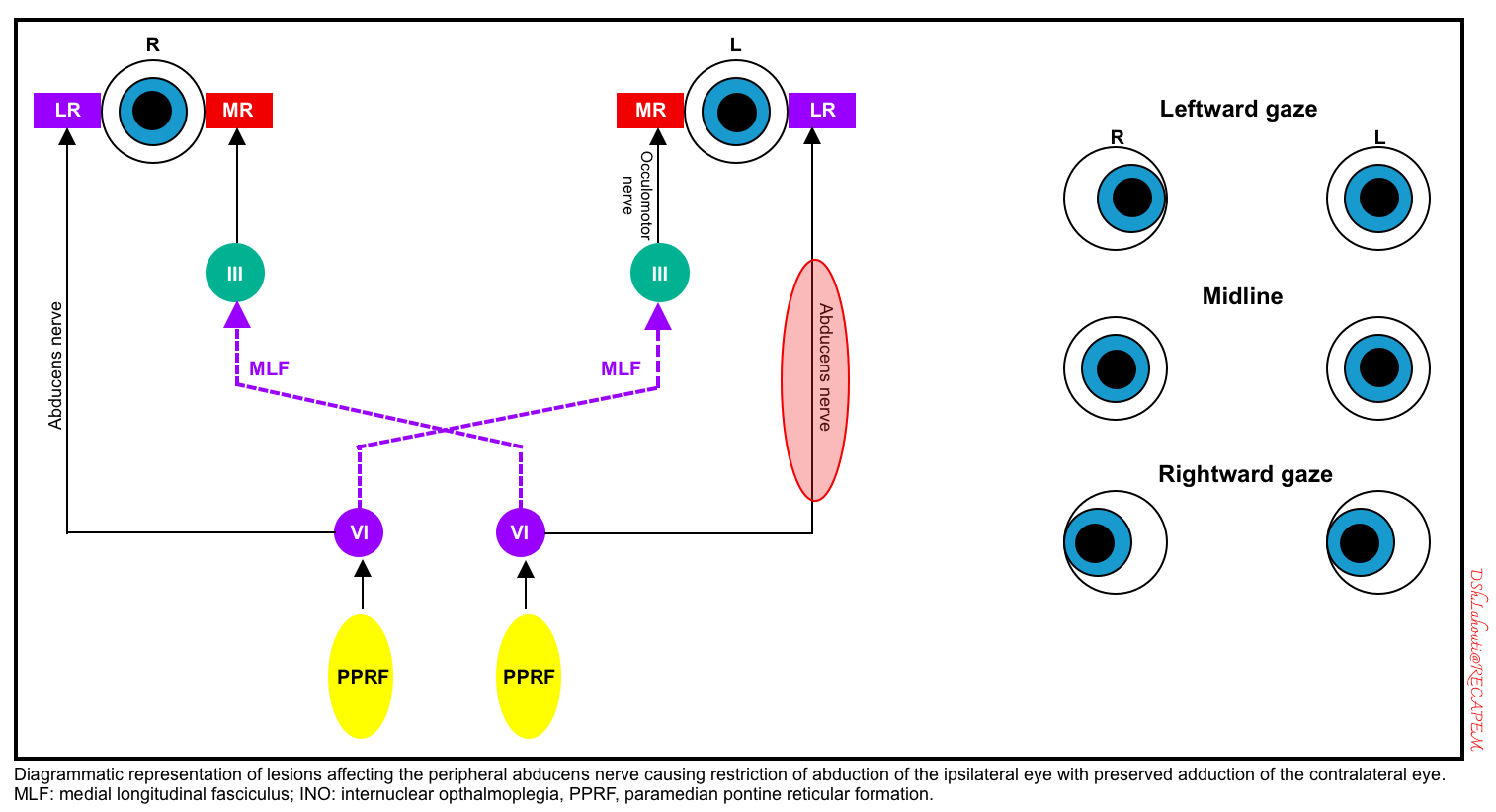
Lateral gaze palsy (damage to CN6-abducens nucleus or PPRF)
- Damage to the abducens nucleus in the pons causes an inability to gaze at all in the ipsilateral direction (i.e. both an inability to abduct the ipsilateral eye and an inability to adduct the contralateral eye).
- Unlike frontal lobe lesions, pontine lesions cause gaze paralysis, which cannot be overcome by the oculocephalic reflex or cold caloric stimulation.
- Damage to the PPRF (paramedian pontine reticular formation) would cause an identical pattern of abnormalities in response to volitional eye movements triggered via the frontal eye fields. However, PPRF damage wouldn’t be expected to affect the oculocephalic or vestibulo-ocular reflexes.
- A schematic diagram of the left abducens nucleus or left PPRF is shown below.
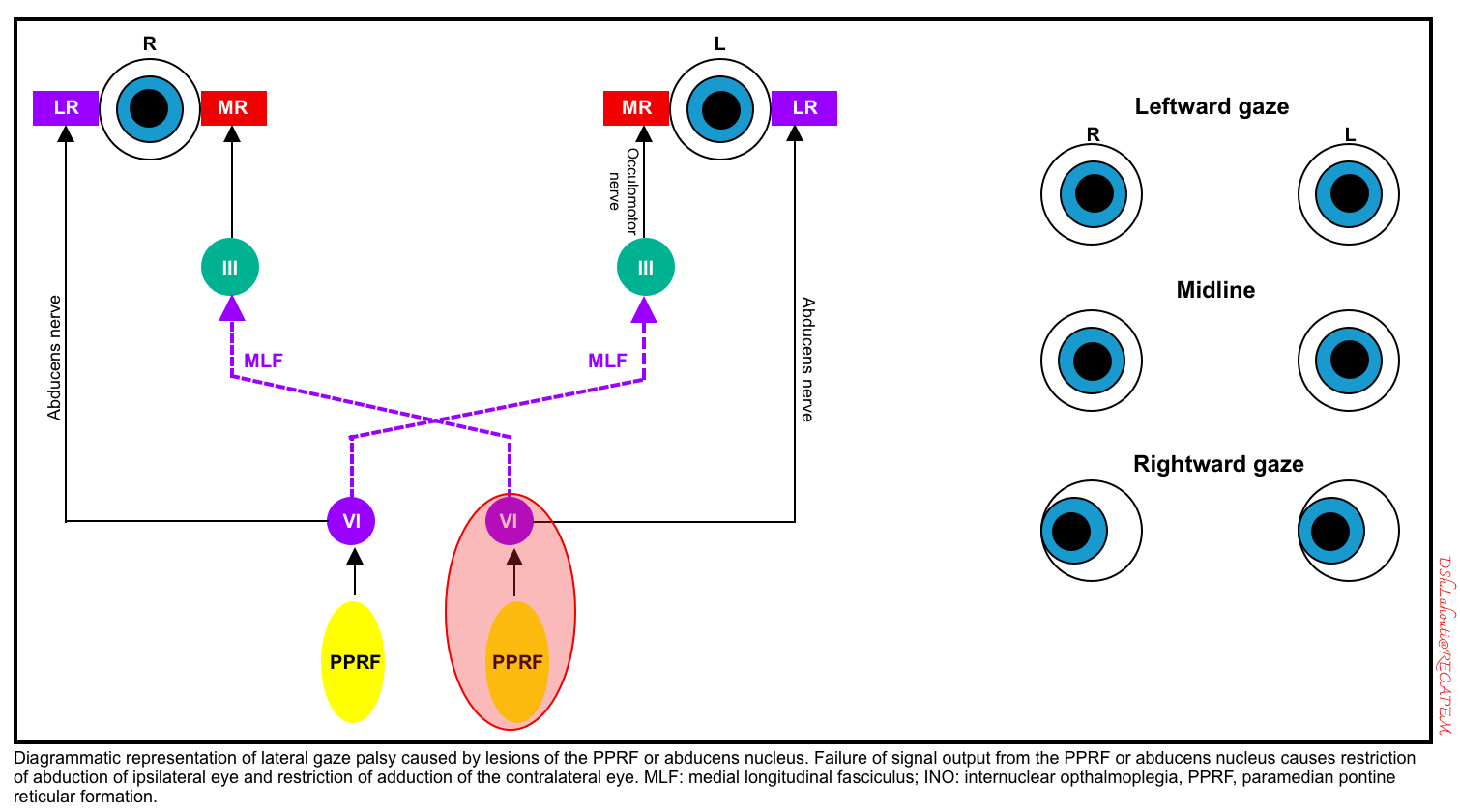
INO (internuclear ophthalmoplegia, due to unilateral MLF damage)
- An internuclear ophthalmoplegia (INO) is caused by a lesion in the (MLF).
- This pathway connects the sixth nerve nucleus on one side up to the third nerve nucleus on the other, allowing for conjugate horizontal gaze.
- A lesion in the MLF is manifested by impaired adduction on the affected side accompanied by nystagmus in the normal eye abducting eye *.
- Convergence (bilateral adduction) is preserved, since this doesn’t depend on the MLF pathway. Preservation of convergence demonstrates that the CN3 and medial rectus muscles are functional, they just are not being triggered properly during horizontal gaze. (However, skew deviation can occur in patients with internuclear ophthalmoplegia, which may make it difficult to test convergence.).
- A schematic diagram of the left MLF is shown below.
- Causes of internuclear ophthalmoplegia include multiple sclerosis, pontine stroke, or tumors.
- Examples of INO: 🎥
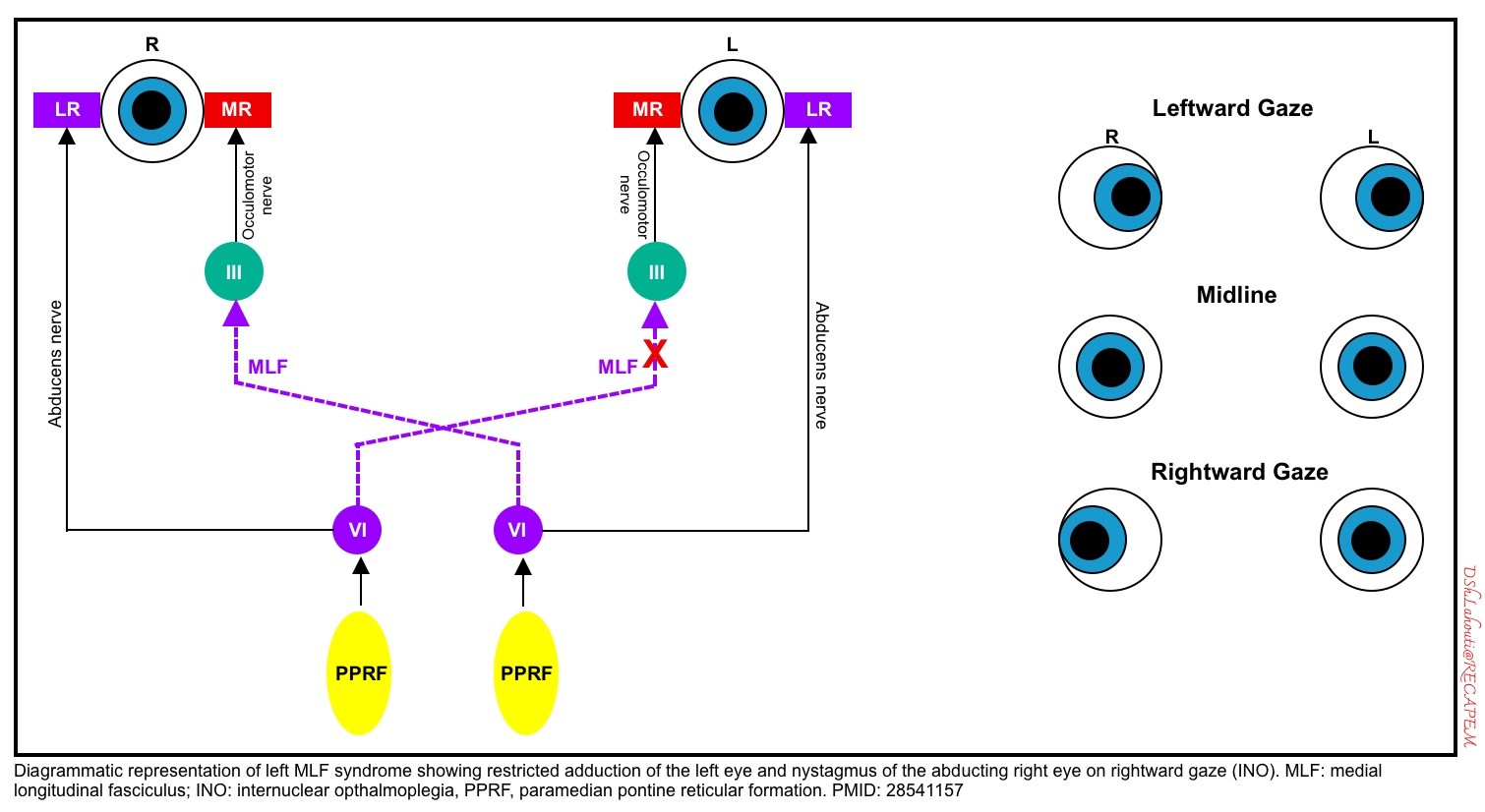
WEBINO (wall-eyed bilateral internuclear ophthalmoplegia)
- WEBINO is due to bilateral MLF damage. A schematic diagram of bilateral MLF lesions is shown below.
- Clinical findings: bilateral adduction deficits, convergence loss, and often a large angle exotropia in primary gaze exist *. 🎥
- Causes:
- Multiple sclerosis, brainstem tumor, or stroke.
- Less common causes: cryptococcus, neuromyelitis optica spectrum disorder.
- Differential diagnosis:
- Bilateral damage to the CN3 nuclei may cause a similar pattern, with the inability of either eye to adduct. However, this would be expected to cause additional abnormalities (e.g. ptosis and pupil dilation).
- Progressive supranuclear palsy may present similarly, but vestibulo-ocular reflexes remain intact.
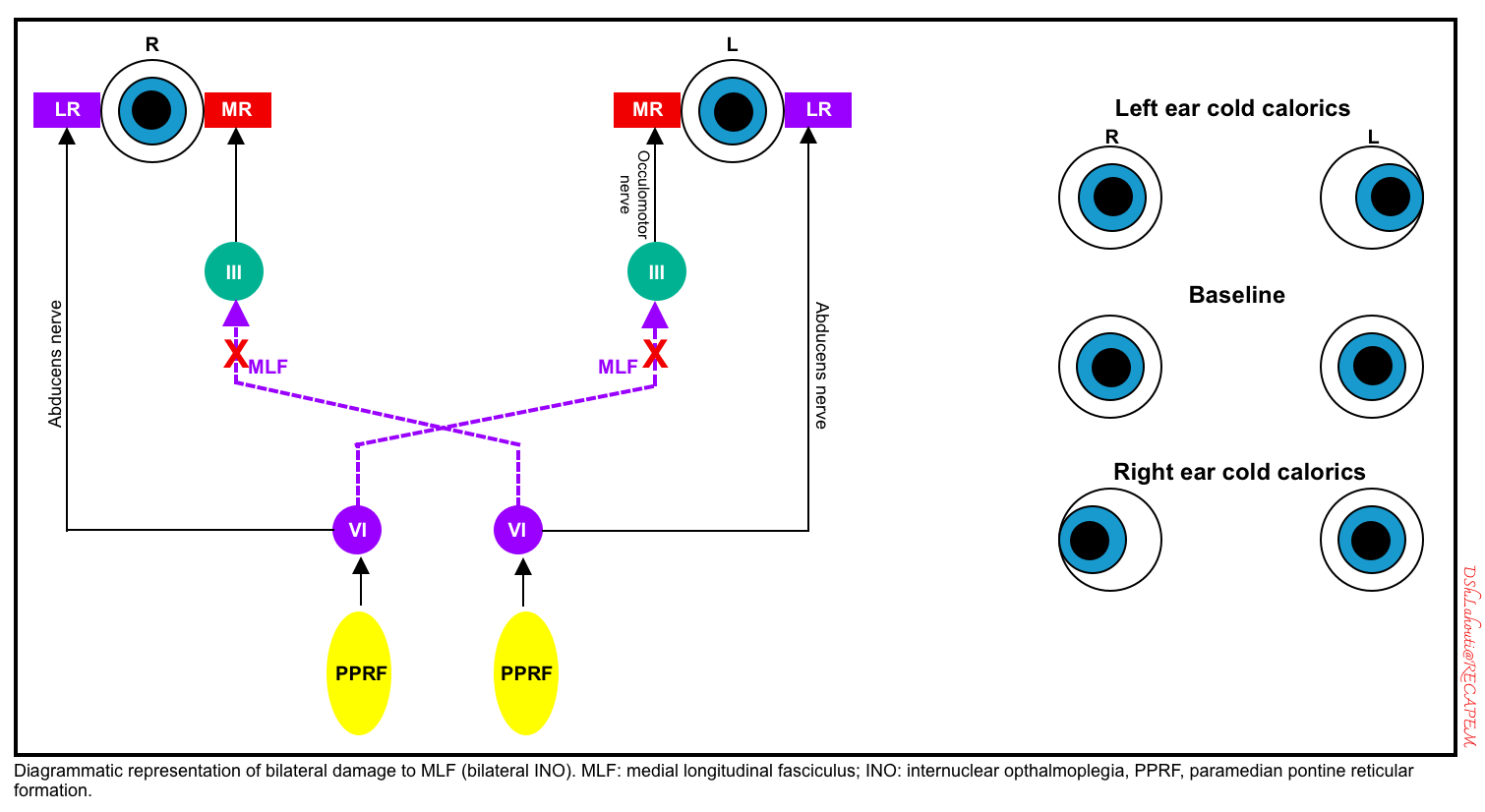
One-and-a-half syndrome
- This occurs due to lesions in the dorsal pontine tegmentum that affect the ipsilateral PPRF or abducens nucleus and ipsilateral MLF.
- Clinical findings
- Lateral gaze palsy in the ipsilateral direction (the affected side) and INO in the contralateral gaze (figure below). 🎥
- Patients often have ipsilateral facial weakness, due to involvement of the nearby facial nerve (CN7) nucleus *. This may be termed an “eight-and-a-half” syndrome (i.e., 7+1.5 = 8.5).
- A schematic diagram of the left-sided lesion is shown below.
- Lateral gaze palsy in the ipsilateral direction (the affected side) and INO in the contralateral gaze (figure below). 🎥
- Causes:
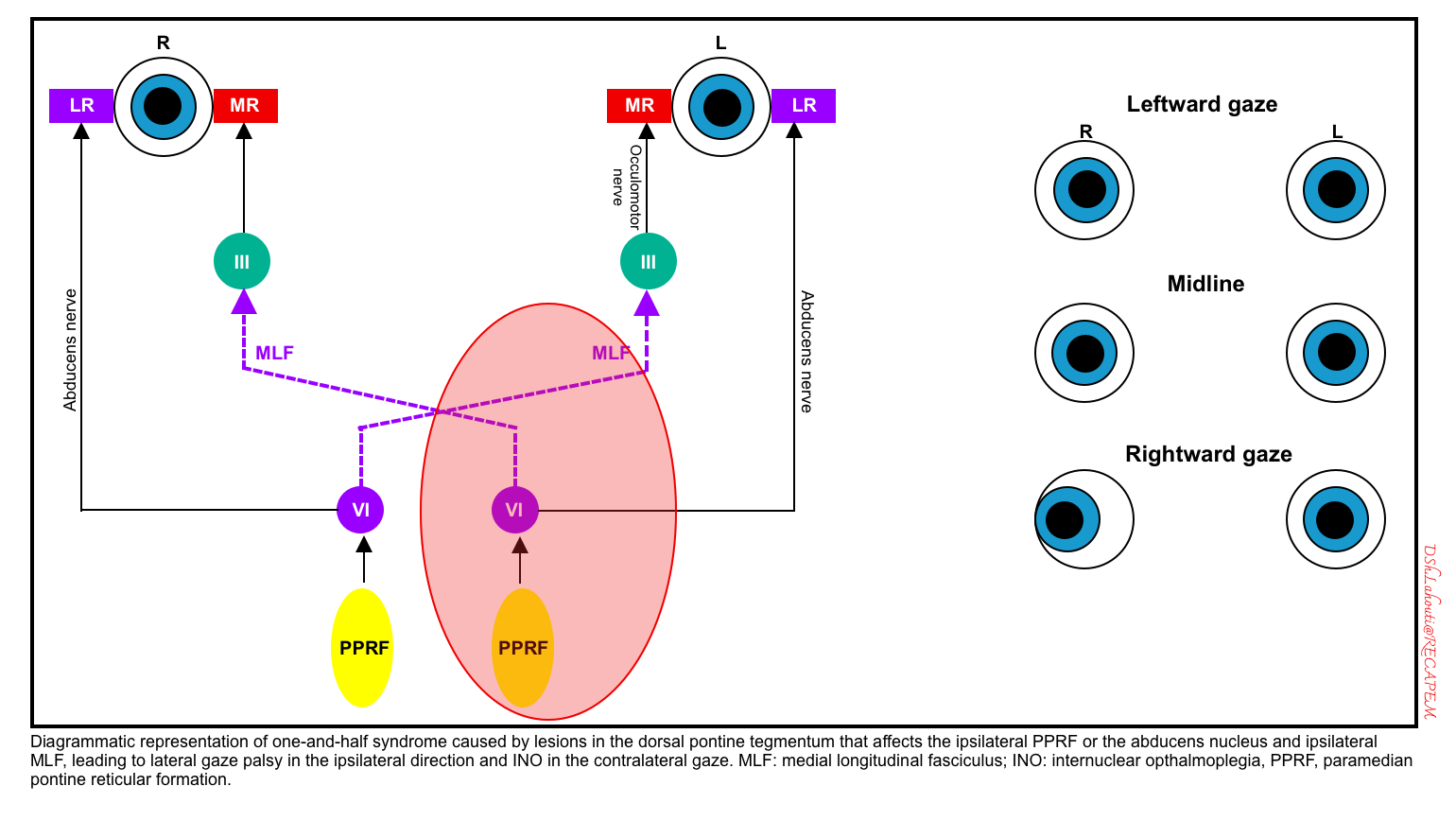
Other abnormal eye movements
▪️Skew deviation (one eye looks up, the other eye looks down)
- Possible causes include:
- Unilateral cerebellar lesion.
- Unilateral brainstem lesion (usually involving the CN8 nucleus).
- Asymmetric dysfunction of the vestibular system causes the brain to think the head is tilted when it is not.
- In the context of a comatose patient, skew deviation may suggest a basilar artery occlusion.
▪️Downward gaze
- This can be caused by
- Bilateral hemispheric dysfunction (e.g. anoxic injury, metabolic coma).
- Thalamic injury (e.g. hemorrhage).
- Dorsal midbrain dysfunction (Parinaud syndrome). Hydrocephalus is a common cause.
▪️Upward gaze
- Possible causes include:
- Bihemispheric damage (e.g. following severe anoxic injury).
- Seizures.
- Brainstem injury.
- Oculogyric crisis. 🎥
▪️Horizontal conjugate roving (ping-pong) eye movements
- Refers to spontaneous, synchronized movements of the eyes back and forth. 🎥
- These are often seen in patients with a reduced level of consciousness.
- These movements reveal the normal function of the midbrain and pons.
- Causes
- Bilateral cerebral hemispheric dysfunction.
- 💡In the context of a coma, horizontal conjugate eye movements exonerate the brainstem, thereby implying that the coma is due to bilateral cerebral hemispheric dysfunction (e.g. a toxic/metabolic coma). Severe bilateral hemispheric infarction can also cause this.
- Cerebellar vermis lesions (but such lesions in isolation would not be expected to cause coma)*.
- Bilateral cerebral hemispheric dysfunction.
▪️Ocular bobbing & dipping
- Bobbing (rapid down, slow up) suggests a pontine lesion.🎥
- Dipping (slow down, rapid up) might suggest bihemispheric dysfunction (e.g. anoxia or a metabolic disorder) *. 🎥
- However, ocular dipping may be caused by a variety of lesions, so it lacks definitive localizing value.
- Note that although these have both fast and slow phases, they are more irregular than nystagmus.
▪️Opsoclonus
- Opsoclonus is a dyskinesia marked by rapid, involuntary, chaotic, multidimensional conjugate eye movements without inter-saccadic intervals. 🎥
- Differential diagnosis of opsoclonus:
- Ocular flutter is similar, but ocular flutter occurs only in the horizontal direction *. 🎥
- Nystagmus may appear similar, but nystagmus involves both a fast (saccadic) phase and a slow phase.
- OMS (opsoclonus-myoclonus syndrome) is associated with paraneoplastic syndromes or viral infection (opsoclonus combined with myoclonus and/or ataxia, encephalopathy, and generalized tremor) 🎥.
- Causes of opsoclonus
- Toxicologic
- Amitriptyline, barbiturates, benzodiazepines, ketamine, lithium, organophosphates, phencyclidine, phenytoin, salicylates, sympathomimetics (amphetamines, cocaine, serotonin syndrome), toluene, venlafaxine.
- Parainfectious
- Often seen in younger patients, may be associated with truncal myoclonus and ataxia.
- Causes include HIV, enterovirus, mumps, Zika virus, CMV, and COVID-19.
- Paraneoplastic
- Often seen in older patients; causes include small cell lung cancer, breast cancer, and ovarian cancer.
- Structural lesion
- Stroke (may occur as a component of lateral medullary syndrome).
- Brain tumor.
- Brainstem encephalitis.
- Demyelinating disorders (including multiple sclerosis, neuromyelitis optica spectrum disorders, and MOG-IgG associated).
- Traumatic brain injury.
- Metabolic processes
- Wernicke encephalopathy.
- Hyperosmolar hyperglycemic state
- Toxicologic
Nystagmus
▪️Background
- It is defined by rhythmic, abnormal eye movements with a “slow” eye movement driving the eye off the target followed by a second movement that brings the eye back to the target.
- The movement can be horizontal, vertical, torsional, or a combination of these movements.
- Nystagmus can be jerky (named for the fast phase) or pendular (slow sinusoidal oscillation to and fro).
- It can be worsened or improved by gaze position, fixation, or covering one eye (latent).
- Nystagmus should be differentiated from “saccadic oscillations”, which are defined as fast, back-to-back (without intersaccadic interval) eye movements driving the eye off the visual target.
Types of nystagmus
▪️Central nystagmus (often due to brainstem pathology)
- Features
- Types and corresponding causes
- Downbeat nystagmus (usually accentuated by downward-lateral gaze). 🎥
- Cervicomedullary junction or cerebellar lesions; or less often pontine or medullary lesions (e.g. tumor, stroke, demyelination) *.
- Wernicke encephalopathy.
- Paraneoplastic.
- Brainstem encephalitis
- Nutritional deficiency: Vitamine B12, thiamine, and magnesium deficiency.
- Hydrocephalus
- Antibody to glutamic acid decarboxylase (GAD) *
- Drugs/intoxication: Lithium, phenytoin, carbamazepine, alcohol, toluene, phencyclidine (PCP), ketamine, amiodarone, opioids *.
- Upbeat nystagmus (usually accentuated by an upward gaze) 🎥.
- Medullary lesions, or less commonly midbrain lesions (e.g., stroke, demyelination).
- Wernicke encephalopathy.
- Paraneoplastic disorders.
- Periodic alternating nystagmus (strictly horizontal, conjugate, jerk nystagmus that periodically alternates its direction of fast phase 🎥)
- Torsional nystagmus 🎥
- Acquired pendular nystagmus (symmetrically oscillating eye movements with no dominant direction, and no corrective phase 🎥).
- The lesion is usually located at the paramedian pontine tract.
- Acute brainstem stroke or with pontine cavernous angioma
- Demyelination, e.g. multiple sclerosis, Toluene abuse *.
- Brainstem stroke
- Whipple’s disease
- Drugs: Phenytoin
- The lesion is usually located at the paramedian pontine tract.
- Convergence-retraction nystagmus: See Parinaud’s syndrome above. 🎥
- Gaze-evoked, direction-changing nystagmus (e.g. right-beating when looking right; left-beating when looking left).
- Chiari I malformation.
- Cerebellar lesions e.g. stroke, demyelination, tumors *
- Wernicke encephalopathy
- Antiepileptic agents, lithium, alcohol, sedatives.
- Downbeat nystagmus (usually accentuated by downward-lateral gaze). 🎥
▪️Peripheral nystagmus
- Features
- May be associated with ear pathology (e.g. hearing loss, tinnitus).
- No features of additional brainstem pathology.
- It is usually suppressed by the visual fixation on an object *.
- This is in contrast to central nystagmus which is not overcome by fixation.
- Can be triggered by head movement.
- The direction of the nystagmus is generally the same (with the fast phase towards the normally functional side).
- However, vestibular disease may cause direction-changing positional nystagmus in supine patients, wherein the direction of the nystagmus varies depending on which ear is facing downward.
- Generally, the peripheral vestibular nystagmus follows either a horizontal or a combination of horizontal and rotatory (horizontal-torsional) pattern, which is in contrast to the purely vertical or torsional nystagmus seen in central vestibular nystagmus *.
- Nystagmus is most marked when looking away from the lesion (looking in the direction of the fast phase).
- Etiology
- Peripheral nystagmus is caused by lesions of the semicircular canals within the ear or CN8-vestibulocochlear (e.g. Meniere’s disease, vestibular neuronitis, benign paroxysmal positional vertigo).
Trigeminal nerve: CN5
▪️Function
- Facial sensation.
- Muscles of mastication, tensor tympani (each trigeminal nucleus is innervated bilaterally by the motor cortex, so unilateral lesions in the motor cortex usually cause no deficit in jaw movement)
▪️Reflexes
- The V1 division of the trigeminal nerve is involved in the corneal reflex.
- The jaw jerk reflex involves purely CN5-trigeminal (which mediates both afferent and efferent limbs). 🎥
- The jaw jerk is normally absent or minimal. A brisk jaw jerk reflex suggests bilateral upper motor neuron pathology, above the level of the trigeminal motor nuclei (as may be seen in amyotrophic lateral sclerosis or diffuse white matter disease)
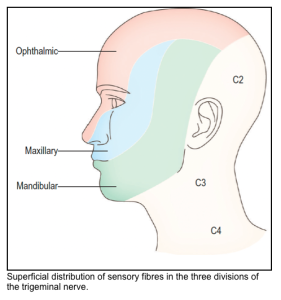
Fascial nerve: CN7
▪️Function
- The facial nerve contains sensory, motor, and parasympathetic components.
- The sensory fibers of the facial nerve supply taste sensation from the anterior two-thirds of the tongue, the floor of the mouth and palate, and also cutaneous sensation from part of the external ear.
- Motor fibers are distributed to the muscles of facial expression, platysma, stylohyoid, the posterior belly of the digastric muscle, and the stapedius muscle of the middle ear.
- The facial nerve is involved in the efferent limb of the corneal reflex.
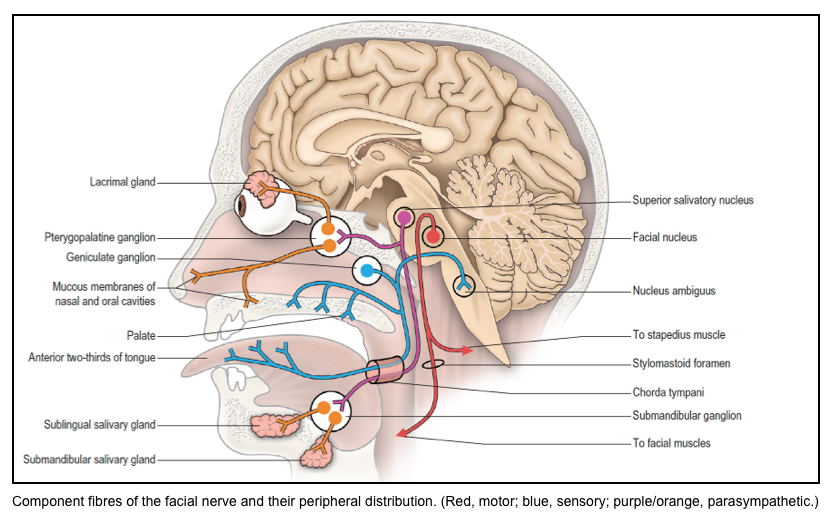
Fascial weakness
▪️Upper motor neuron lesions
- Clinical effects:
- Weakness predominantly involves the lower face. (The forehead receives bilateral innervation from both cortical hemispheres, so it is spared.)
- Emotional expression (e.g., spontaneous smiling) may be preserved because the frontal lobe and extrapyramidal systems also provide stimulation to the facial nucleus. However, patients may be unable to smile upon command!
- Caused by any lesion within the corticobulbar tract that lies above the facial nucleus (e.g. the motor cortex).
▪️Lower motor neuron lesions (fascial nerve or fascial nucleus in brainstem)
- Clinical effects:
- Weakness of the entire ipsilateral face.
- Hyperacusis.
- Causes:
- A lower motor neuron lesion usually indicates a lesion within the facial nerve (e.g. Bell’s palsy). More on this here.
- However, a lower motor neuron lesion can involve the cranial nerve nucleus, or the nerve fascicle as it exits the brainstem; so this can occur in the context of brainstem pathology. Lesions of the facial nucleus usually localize to the posterior, and inferior pons. 🎥
Corneal reflex
▪️Neuroanatomy
- The afferent limb arises from small unmyelinated pain fibers in the cornea and is mediated by ophthalmic division of the trigeminal nerve (V1).
- The afferent limb (CN V) activates the dorsal parts of both ipsi-and contra-lateral facial nuclei in the pons.
- The efferent limb of the reflex occurs via CN7-facial.
- Therefore, stimulating one eye should elicit blinking bilaterally.
- There are also connections with the oculomotor nucleus so that the eyeballs move upward in concert with lid closure (therefore, we are unable to observe this).
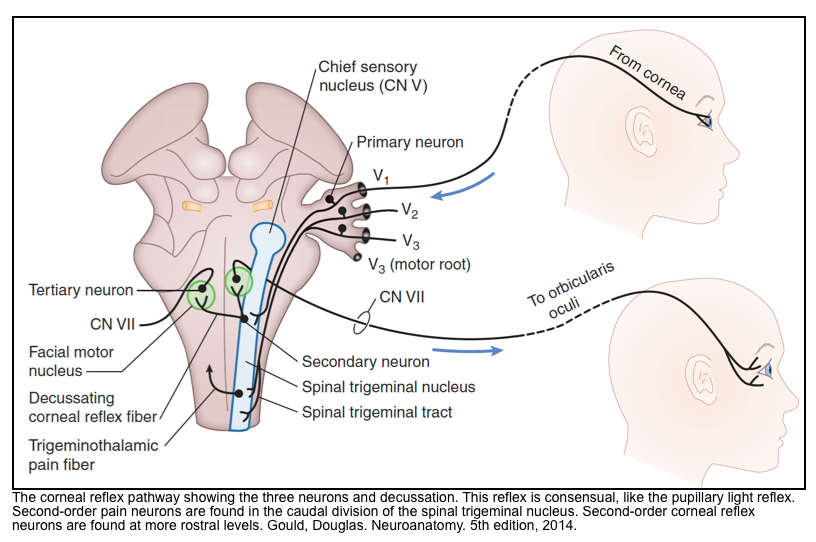
▪️How to test for corneal reflex?
- The corneal reflex is tested by gently touching the edge of the cornea with a rolled tissue or cotton swab and observing the responsive blink 🎥. As an alternative, squirts of water or saline are less likely to scratch the cornea in repeated assessments.
▪️Interpretation
- An acute cerebral lesion can cause contralateral suppression of the corneal reflex.
- Unilateral pontine injuries may cause an ipsilateral loss of the corneal reflex.
- If the contralateral eye blinks but the ipsilateral eye does not, this indicates an ipsilateral facial nerve (CN7) paralysis.
- Bell’s phenomenon
- Conjugate upward deviation of the eyes without eyelid closure.
- Normally the eyes often elevate when they close, but we are unable to observe this. Seeing the eyes elevate indicates intact sensation (CN5) and intact pathways to CN3 (to elevate the eye), with dysfunction of the eyelid (CN7); essentially revealing a CN7 palsy.
- Corneomandibular reflex (aka corneal pterygoid reflex or Wartenberg reflex) 🎥
- The jaw deviates away from the side of corneal stimulation, along with bilateral eye blinking.
- Implies a structural lesion injuring the trigeminal nucleus above the mid-pons level. Potential causes include herniation, intrinsic upper brainstem lesions, amyotrophic lateral sclerosis, or multiple sclerosis.
⚠️The corneal reflex may also be absent in the following conditions:
- Deep metabolic or toxic coma
- Deep sedation
- Corneal reflexes may also be reduced or absent at baseline in older or diabetic patients.
🔴Absent corneal reflexes 24 hours after cardiac arrest is usually, but not invariably, an indication of poor prognosis (assuming the patient has not been sedated).
Glossopharyngeal nerve (CN9) and Vagus nerve (CN10)
▪️Background
- CN9 and CN10 generally function as a single entity.
- CN9 and CN10 lie in very close proximity, with disease processes often involving both nerves. For example, motor fibers of both nerves originate from the nucleus ambiguus in the medulla.
- It’s difficult to separately examine CN9 vs. CN10 during a basic neurologic examination since their functions overlap.
- For general clinical purposes, it’s reasonable to consider CN9/10 as a single functional entity.
▪️Function of CN 9&10
- Pharyngeal sensation and musculature (swallowing).
- Laryngeal muscles (speaking, mostly via the recurrent laryngeal nerve which is a branch of CN10).
- Aortic arch and carotid artery baroreceptors.
- CN10 provides parasympathetic innervation to the heart, lungs, and upper gastrointestinal tract.
▪️Clinical examination of CN 9&10
- Soft palate movement with phonation (saying “ahh”).
- Symmetric upward movement of the uvula suggests intact function of CN9/10.
- Unilateral damage to CN9/10 causes the uvula to be pulled towards the normal side.
- Bilateral damage to CN9/10 causes the uvula to remain immobile; this may correlate with dysphagia (especially with liquids).
- Voice:
- CN10 innervates the vocal cords.
- Lesions to CN10 may be suggested by a breathy, nasal, or hoarse voice.
- ⚠️However, dysarthria may result from abnormalities in CN 5, 7, 9, 10, or 12; so this isn’t necessarily specific to a lesion of CN10.
- Cough reflex (among intubated patients):
- CN10 vagus is required for the afferent pathway of the cough reflex.
Cough reflex
▪️Cough reflex basics
- The cough reflex involves the vagus nerve (CN10, near the medulla).
- This reflex will be preserved in most coma states (which usually involve higher brain centers).
- Reliably eliciting the cough reflex requires an intubated patient, in whom endobronchial stimulation can be applied by suctioning the endotracheal tube.
- The cough reflex is one of the most durable reflexes, which is generally preserved even in severe brain injury.
- For example, following anoxic brain injury, lack of a cough reflex after 24 hours carries a likelihood ratio of 85 for poor neurologic outcome.
▪️Etiology of the absent cough reflex
- Damage to the medulla (e.g. tonsillar herniation, brain death).
- Extremely profound toxic/metabolic coma (e.g. barbiturate or baclofen overdose).
- Neuromuscular paralysis (e.g. lingering effects of rocuronium used for intubation).
💡When encountering a patient who lacks a cough reflex without any obvious cause, always consider the possibility of a lingering neuromuscular paralytic agent. If the patient otherwise appears paralyzed (e.g. absent deep tendon reflexes), this should be immediately evaluated at the bedside using a peripheral nerve stimulator.
Spinal accessory nerve: CN11
▪️Neuroanatomy
- CN11 is a motor nerve that innervates the trapezius (which raises the shoulder) and the sternocleidomastoid muscles (which turns the head to the opposite side).
- 👉Contraction of one sternocleidomastoid (SCM) turns the head, whereas contraction of both (SCM) is involved in head flexion.
- Unilateral upper motor neuron lesion in the cortex causes contralateral weakness of the trapezius, with relative sparing of sternocleidomastoid strength.
- Similar to the upper facial muscles, the sternocleidomastoid muscle is controlled by the bilateral cerebral hemispheres. However, a focal seizure tends to cause contralateral neck flexion, suggesting that dominant control of each sternocleidomastoid muscle comes from the ipsilateral cerebral hemisphere (Berkowitzv 2022).
▪️Clinical examination
- Trapezius strength is assessed by asking the patient to shrug their shoulders.
- Sternocleidomastoid strength may be assessed by asking the patient to turn their head in both directions. For a supine patient, lifting their head off the pillow requires the bilateral sternocleidomastoid muscles.
▪️Neck flexion weakness
- Bilateral weakness of the sternocleidomastoid muscles causes weakness of head flexion (e.g. the patient may be unable to lift their head off the pillow).
- Common causes include myasthenia gravis, myopathy, and Guillain-Barre syndrome.
- Weakness of neck flexion may tend to track with respiratory muscle weakness. This could reflect that they share the same segmental innervation *.
Hypoglossal nerve: CN 12
▪️Neuroanatomy
- CN 12 (hypoglossal nerve) is a pure motor nerve innervating all muscles of the tongue except the palatoglossus (which is innervated by CN 10).
- The hypoglossal nuclei reside in the medulla, and as with other pure motor cranial nerves that innervate skeletal muscle, these nuclei are dorsal/posterior and midline.
- Just as with CN 7, each CN 12 nucleus receives input from the corticobulbar tracts originating in the contralateral motor cortex (these are the upper motor neurons in the system; the bilateral CN 12s contain the lower motor neurons).
- All tongue muscles are bilaterally innervated except genioglossus, which is the main tongue protruder, and is only innervated by the contralateral hemisphere.
▪️Clinical examination
- Unilateral tongue weakness causes the tongue to deviate to the weak side (when trying to protrude the tongue midline, the stronger side wins, pushing the tongue over to the weaker side).
- A unilateral hypoglossal nucleus, fascicle, or nerve lesion results in ipsilateral tongue weakness, causing deviation of the tongue toward the side of the lesion.
- A lesion of the corticobulbar tract (in the cortex, subcortical white matter, or brainstem superior to the hypoglossal nucleus in the medulla) results in contralateral tongue weakness, causing deviation of the tongue away from the side of the lesion. Why?
- All tongue muscles are bilaterally innervated except genioglossus, the main tongue protruder, which is only innervated by the contralateral hemisphere. So the main muscle affected by a hemispheric lesion is the contralateral genioglossus, causing the tongue to protrude toward the weak side (overcome by the strong genioglossus on the normal side).
- ⭐️Weakness of the tongue can cause dysarthria, specifically for lingual sounds (e.g., difficulty producing the consonants “L,” “D,” and “T”).
▪️Causes of CN12 dysfunction
- Unilateral tongue weakness
- Lower motor neuron disease
- Internal carotid dissection.
- Medial medullary syndrome (Dejerine syndrome).
- In medial medullary syndrome (caused by infarct in the territory of the anterior spinal artery), the CN 12 fascicle (portion of the nerve still in the brainstem) and the descending (not-yet-crossed) corticospinal tract are affected, so there will be ipsilateral tongue weakness and contralateral body weakness.
- Upper motor neuron disease
- A lesion of the corticobulbar tract (in the cortex, subcortical white matter, or brainstem superior to the hypoglossal nucleus in the medulla)
- Lower motor neuron disease
- Bilateral tongue weakness with atrophy and fasciculations can be seen in motor neuron disease such as ALS.
Motor system
Neuroanatomy
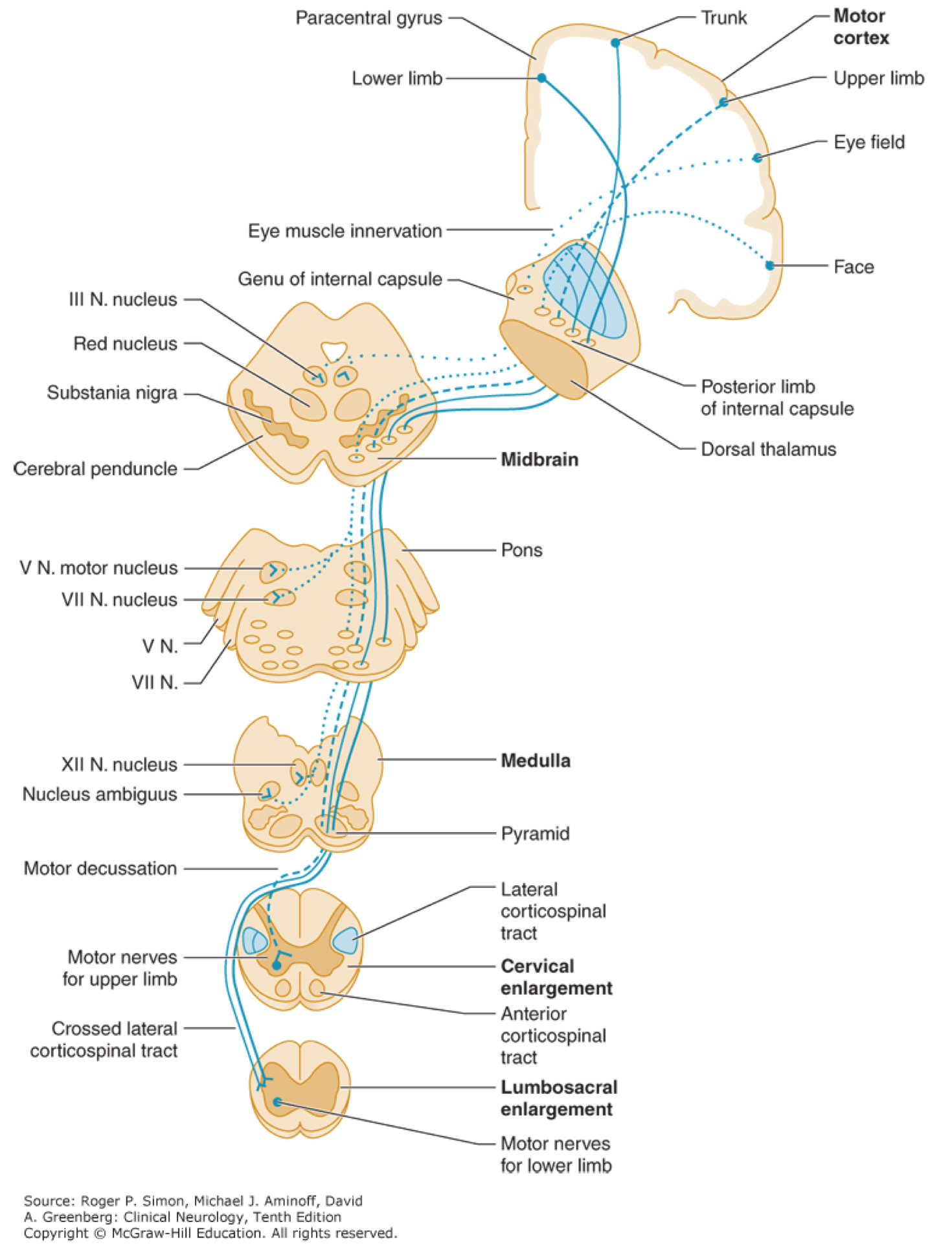
▪️Corticospinal tract (pyramidal tract)
- Upper motor neuron cell bodies lie in the motor cortex (within the precentral gyrus).
- Their axons travel in the subcortical white matter within the posterior limb of the internal capsule.
- They run through the cerebral peduncles into the ventral/anterior brainstem.
- They cross (decussate) at the junction of the medulla and the cervical spinal cord.
- They run down the posterolateral spinal cord.
- Finally, upper motor neuron axons synapse onto lower motor nerves within the anterior horn of the spinal cord grey matter.
▪️Corticobulbar tract
- Primarily involved in carrying the motor function of the non-oculomotor cranial nerves (e.g. cranial nerves V, VII, IX, XII, and motor region of cranial nerve X.
- The corticobulbar tract originates in the primary motor cortex of the frontal lobe, just superior to the lateral fissure and rostral to the central sulcus in the precentral gyrus.
- It descends via corona radiata and the genue of the internal capsule with a few fibers in the posterior limb of the internal capsule.
- In the midbrain, the internal capsule becomes the cerebral peduncle.
- The corticobulbar fibers exit at the appropriate level of the brainstem to synapse on the lower motor neurons of the cranial nerves ( V, VII, IX, XII, the motor regions of cranial nerve X in the nucleus ambiguus.
- The corticobulbar tract innervates cranial motor nuclei bilaterally except for the lower facial nuclei (which innervates facial muscles below the eyes) and the genioglossus muscle, which are innervated only unilaterally by the contralateral cortex.
Motor exam
▪️Assess muscle strength 🎥, tone 🎥 , pronator drift 🎥.
- Localizing the weakness 👉 here.
🔴Causes of increased motor tone or rigidity
- Intoxication (e.g. serotonin syndrome, neuroleptic malignant syndrome, malignant hyperthermia).
- Withdrawal of Parkinson’s medications.
- Seizure.
- Upper motor neuron lesion (involving the corticospinal tract).
- Basal ganglia dysfunction.
- Bilateral frontal lobe dysfunction may cause paratonic rigidity.
🔴Causes of flaccidity
- Acute upper motor neuron lesion (especially spinal cord injury); may initially cause flaccidity, before the development of spasticity. This may occur in the context of spinal shock.
- Lower motor neuron lesion.
- Intoxication.
- Chemical paralysis.
Deep tendon reflexes
▪️Reflexes: The Neuroanatomy of commonly tested reflexes:
- Biceps: C5-C6.
- Brachioradialis: C6.
- Triceps: C7.
- Patellar: L4.
- Achilles: S1.
▪️Evaluation 🎥
- Standard numeric scale:
- 0 = Absent.
- 1+ = Reduced, or seen only on reinforcement.
- 2+ = Normal.
- 3+ = Brisk.
- 4+ = Hyperreflexia associated with unsustained clonus.
- 5+ = Hyperreflexia with sustained clonus.
- Deep tendon reflexes are normal if they are 1+, 2+, or 3+ as long as they are symmetric and there isn’t a dramatic difference between arms versus legs.
- The spreading of reflexes to muscles that aren’t being tested is a reflection of hyperreflexia.
Clonus
▪️Background
- It may be conceptualized as a form of profound hyperreflexia, wherein each muscle contraction triggers another reflexive contraction.
- Nonsustained clonus (<5-10 beats) that is symmetric may be normal. However, sustained clonus (>5-10 beats) is always abnormal. Unfortunately, there is some confusion about how to define “sustained clonus,” with some authors using this to refer to clonus that occurs indefinitely.
- As part of the physical examination, ankle clonus is generally tested. 🎥
- However, clonus may occur at other joints as well (e.g. it could be inadvertently triggered while testing a patellar reflex). 🎥
- Spontaneous clonus is triggered by minor movement, leading to rhythmic, large muscle contractions. This may be confused with seizures.
▪️Etiology
- Upper motor neuron dysfunction as spasticity develops (e.g. due to stroke, trauma, cerebral palsy, or multiple sclerosis).
- Toxicologic: Toxicological clonus may be suggested by symmetric clonus and diffuse hyperreflexia (without any focal neurological signs).
- Serotonin syndrome is the most common toxicological association. Clonus due to serotonin syndrome is usually most prominent in the legs.
- Anticholinergic toxicity.
- Baclofen withdrawal.
- Other
- Preeclampsia.
- Paraneoplastic or autoimmune encephalopathies.
- Sympathetic hyperactivity due to various etiologies.
Babinski sign
▪️Babinski sign 🎥
▪️Clinical significance
- A Babinski sign in an adult is always abnormal and indicates an upper motor neuron disease.
- A unilateral Babinski sign indicates pathology somewhere within the corticospinal tract (contralateral cerebral hemisphere, contralateral brainstem, or ipsilateral spinal cord).
- A bilateral Babinski sign:
- In isolation, this often suggests spinal cord pathology.
- A bilateral Babinski sign may result from the brainstem or cerebral hemisphere dysfunction (e.g. due to bilateral brain injury, general anesthesia, coma from organ failure, or seizure).
Hoffmann sign
▪️Definition: Flicking the third digit causes flexion of the first and second digits. 🎥
▪️Clinical significance
- Presence of Hoffmann sign is indicative of upper motor neuron disease.
- Presence of bilateral and symmetric Hoffmann sign may be seen in normal individuals.
Coordination
▪️Background
- Contrary to popular belief, poor performance on the above tests doesn’t necessarily indicate a cerebellar lesion. Instead, it may reflect a pathology involving the cerebellum, proprioception, vision, basal ganglia, or motor pathways.
- Cerebellar pathology is likely only if abnormalities in other subsystems have been excluded (e.g. adequate vision, intact strength, normal reflexes, intact proprioception, and absence of other features of basal ganglia dysfunction).
▪️Evaluation
- Gait (this often cannot be tested in critically ill patients): 🎥
- Extremity coordination 🎥
- Finger-nose-finger test.
- Rapid alternating movements (dysdiadochokinesia).
Involuntary movement
🔴Tremor
- Common causes in critical care settings include
- Structural lesions:
- Cerebellar lesion (intention tremor).
- Midbrain lesion (postural tremor).
- Medication-induced:
- Beta-agonists.
- Dopamine antagonists.
- Valproate.
- Amiodarone.
- Cyclosporine, tacrolimus.
- Lithium.
- Withdrawal (e.g. alcohol or opioid).
- Tremors may occur as a component of acute onset parkinsonism.
- Structural lesions:
🔴Myoclonus
- Myoclonus consists of “brief”, sudden, “shock-like movements”, which can originate from active muscle contraction or loss of muscle tone (the latter is called negative myoclonus or asterixis) *.
- Myoclonus is both sudden and brief (“jerk‑like”) when compared to other involuntary movement.
- A tremor discharge may be brief but does not result in a jerk or sudden movement.
- Dystonic spasms or dyskinetic jerks may be sudden, but they are not lightning brief.
- Myoclonus is both sudden and brief (“jerk‑like”) when compared to other involuntary movement.
- Different forms of myoclonus can vary considerably, for example:
- Synchronized, dramatic jerking of multiple limbs (e.g. post-anoxic myoclonus).
- Asynchronous, multifocal twitching of individual muscles (multifocal myoclonus).
- Diffuse myoclonus without any focal abnormalities on neurological examination is most often due to metabolic abnormalities or medications.
- This is frequently encountered as a component of toxic/metabolic encephalopathy.
- ⭐️Contrary to popular belief, myoclonus and asterixis (“negative myoclonus”) are not specific to hepatic dysfunction.
🔴Asterixis
- It refers to sudden, brief, arrhythmic lapses of sustained posture due to involuntary interruption in muscle contraction.
- This is commonly assessed by asking the patients to hold their hands outstretched as if they were stopping traffic.🎥
- 👉Asterixis may begin after a latent period of 30 seconds, so be cautious about immediately concluding that there is no asterixis.
- Causes *
- Metabolic derangements
- Hepatic encephalopathy (although asterixis may be lost in the most severe stages of hepatic encephalopathy) *.
- Uremic encephalopathy.
- Hypercapnia and/or hypoxemia.
- Hypoglycemia.
- Severe electrolyte abnormalities (⬇️K, ⬇️Mg).
- Urea cycle defects causing hyperammonemia.
- Wilson’s disease.
- Medication
- Antiseizure medications (e.g. phenytoin, valproate, carbamazepine, gabapentin) *.
- Levodopa.
- Opioids.
- Anticholinergics.
- Benzodiazepines.
- Lithium.
- Clozapine.
- Structural lesions (often involving the thalamus)
- Hemorrhage (subdural, subarachnoid, or intraparenchymal).
- Infarction.
- Neoplasia (primary or metastatic).
- Cerebral toxoplasmosis.
- Viral encephalitis.
- Metabolic derangements
🔴Fasciculations
- These are small involuntary muscle contractions that don’t cause movement across a joint.
- Causes of fasciculations *
- Any disease involving the lower motor neurons.
- Electrolyte abnormalities (e.g. ⬇️K, ⬇️Mg).
- Hyperthyroidism.
- Medication side effects (e.g. steroid, acetylcholinesterase inhibitors, isoniazid, bronchodilators, caffeine).
- Benzodiazepine withdrawal.
- Uremia.
- Following brain death.
🔴Paratonic rigidity
- Paratonic rigidity (aka. gegenhalten) is a form of hypertonia marked by involuntary variable resistance to passive movements (especially a quick movement of the arm). Resistance occurs with a force equal to and opposite to that applied by the examiner. This may be misinterpreted as oppositional behavior.
- Causes:
- Frontal lobe disorders, especially bilateral frontal lobe dysfunction.
- Metabolic encephalopathy.
- Catatonia.
Somatosensory system
Neuroanatomy
▪️Pain & temperature
- Unlike the other major tracts, the dorsal root neurons decussate immediately within the spinal cord. Subsequently, second-order neurons ascend within the anterolateral spinal cord.
- It takes 2-3 spinal segments for the fibers to reach the opposite side of the spinal cord. Thus, a lateral cord lesion will affect contralateral pain sensation beginning a few segments below the level of the lesion.
- Eventually, these neurons join the medial lemniscus in the pons and travel together with the dorsal column neurons to the ventral posterolateral nucleus of the thalamus.
- Clinically, pain perception can be measured by determining whether a pin is perceived as feeling sharp.
▪️Vibration & proprioception
- After traveling up the dorsal column of the spinal cord, axons ascend to the posterior column nuclei in the medulla:
- Nucleus gracilis (from the lower body; more medial).
- Nucleus cuneatus (from the upper body; more lateral).
- Neurons from these nuclei decussate in the base of the medulla (similarly to the corticospinal tract), subsequently forming the medial lemniscus.
- The medial lemniscus travels to the VPL (ventral posterior lateral) nucleus of the thalamus, which is also the destination of the anterolateral (spinothalamic) tract.
- Finally, neurons project through the posterior limb of the internal capsule to the primary somatosensory cortex.
Sensory examination
- It is not usually necessary to test both pain and temperature; either will suffice.
- Pain (video here 🎥)
- First explain to the patient that you will be touching each finger with either the sharp or the dull end of a safety pin, and demonstrate each.
- Pain sensation may be evaluated by determining whether the patient can perceive a gentle stimulation from a pin or broken wooden swab as “sharp”
- Temperature 🎥
- It can be tested in a fashion analogous to pain; a reasonable stimulus is the flat portion of a tuning fork after it has been immersed in cold water and dried.
- Pain (video here 🎥)
- Position sense 🎥
- It may be evaluated by determining if the patient can sense very subtle adjustments to the finger or toe position, either upwards or downwards. The digit should be gently grasped from the sides, to avoid providing tactile clues regarding which direction the digit is being moved.
- Vibration sense 🎥
- Vibration sense can be tested by tapping a 128-Hz tuning fork lightly against a solid surface to produce a slight (silent) vibration.
- With the patient’s eyes closed, hold the nonvibrating end of the tuning fork firmly on the distal interphalangeal joint of the patient’s left thumb and ask the patient if the vibration is detectable.
- If this isn’t sensed, then stimulate more proximally until the patient can perceive the vibration. Avoid stimulation over bones, as the bone may transmit vibration proximally.
References
1. PMID: 27907952. Wijdicks EF. Neurology of Critical Care. Semin Neurol. 2016 Dec;36(6):483-491. doi: 10.1055/s-0036-1592108. Epub 2016 Dec 1.
2. PMID: 18533088. Barrett KM, Freeman WD. 71-year-old woman with loss of right-sided vision and cognitive deficits. Mayo Clin Proc. 2008 Jun;83(6):708-11. doi: 10.4065/83.6.708.
3. PMID: 16567710. Zhang X, Kedar S, Lynn MJ, Newman NJ, Biousse V. Homonymous hemianopias: clinical-anatomic correlations in 904 cases. Neurology. 2006 Mar 28;66(6):906-10. doi: 10.1212/01.wnl.0000203913.12088.93.
4. PMID: 32924623. Li B, Sursal T, Bowers C, Cole C, Gandhi C, Schmidt M, Mayer S, Al-Mufti F. Chameleons, red herrings, and false localizing signs in neurocritical care. Br J Neurosurg. 2022 Jun;36(3):298-306. doi: 10.1080/02688697.2020.1820945. Epub 2020 Sep 14.
5. PMID: 23322826. Bae YJ, Kim JH, Choi BS, Jung C, Kim E. Brainstem pathways for horizontal eye movement: pathologic correlation with MR imaging. Radiographics. 2013 Jan-Feb;33(1):47-59. doi: 10.1148/rg.331125033.
6. PMID: 36161185. Wang T, Cao D, Han J. Case report: A variant of wall-eyed bilateral internuclear ophthalmoplegia from unilateral pons infarction. Front Neurosci. 2022 Sep 9;16:974645. doi: 10.3389/fnins.2022.974645.
7. PMID: 28187795. Wijdicks EF. Management of the comatose patient. Handb Clin Neurol. 2017;140:117-129. doi: 10.1016/B978-0-444-63600-3.00008-8.
8. PMID: 18474744. Zaro-Weber O, Galldiks N, Dohmen C, Fink GR, Nowak DA. Ocular flutter, generalized myoclonus, and trunk ataxia associated with anti-GQ1b antibodies. Arch Neurol. 2008 May;65(5):659-61. doi: 10.1001/archneur.65.5.659.
9. PMID: 21601073. Thurtell MJ, Leigh RJ. Nystagmus and saccadic intrusions. Handb Clin Neurol. 2011;102:333-78. doi: 10.1016/B978-0-444-52903-9.00019-4.
10. PMID: 15826997. Tilikete C, Vighetto A, Trouillas P, Honnorat J. Potential role of anti-GAD antibodies in abnormal eye movements. Ann N Y Acad Sci. 2005 Apr;1039:446-54. doi: 10.1196/annals.1325.042.
11. PMID: 35863882. Walters J. Weakness in the intensive care unit. Pract Neurol. 2022 Jul 21:pn-2022-003422. doi: 10.1136/pn-2022-003422. Epub ahead of print.
12. PMID: 20057497. Abdo WF, van de Warrenburg BP, Burn DJ, Quinn NP, Bloem BR. The clinical approach to movement disorders. Nat Rev Neurol. 2010 Jan;6(1):29-37. doi: 10.1038/nrneurol.2009.196.
13. PMID: 27807107. Ellul MA, Cross TJ, Larner AJ. Asterixis. Pract Neurol. 2017 Jan;17(1):60-62. doi: 10.1136/practneurol-2016-001393. Epub 2016 Nov 2.
14. PMID: 36625084. Thabut D, et al. Association Française pour l’Etude du Foie (AFEF) group of experts of HE recommendations. Diagnosis and management of hepatic encephalopathy: The French recommendations. Liver Int. 2023 Apr;43(4):750-762. doi: 10.1111/liv.15510. Epub 2023 Jan 24.
15. PMID: 27907965. Schaefer SM, Rostami R, Greer DM. Movement Disorders in the Intensive Care Unit. Semin Neurol. 2016 Dec;36(6):607-614. doi: 10.1055/s-0036-1592356. Epub 2016 Dec 1.
Add comment