January 14, 2021 by Shahriar Lahouti. Last update 9 April, 2023.
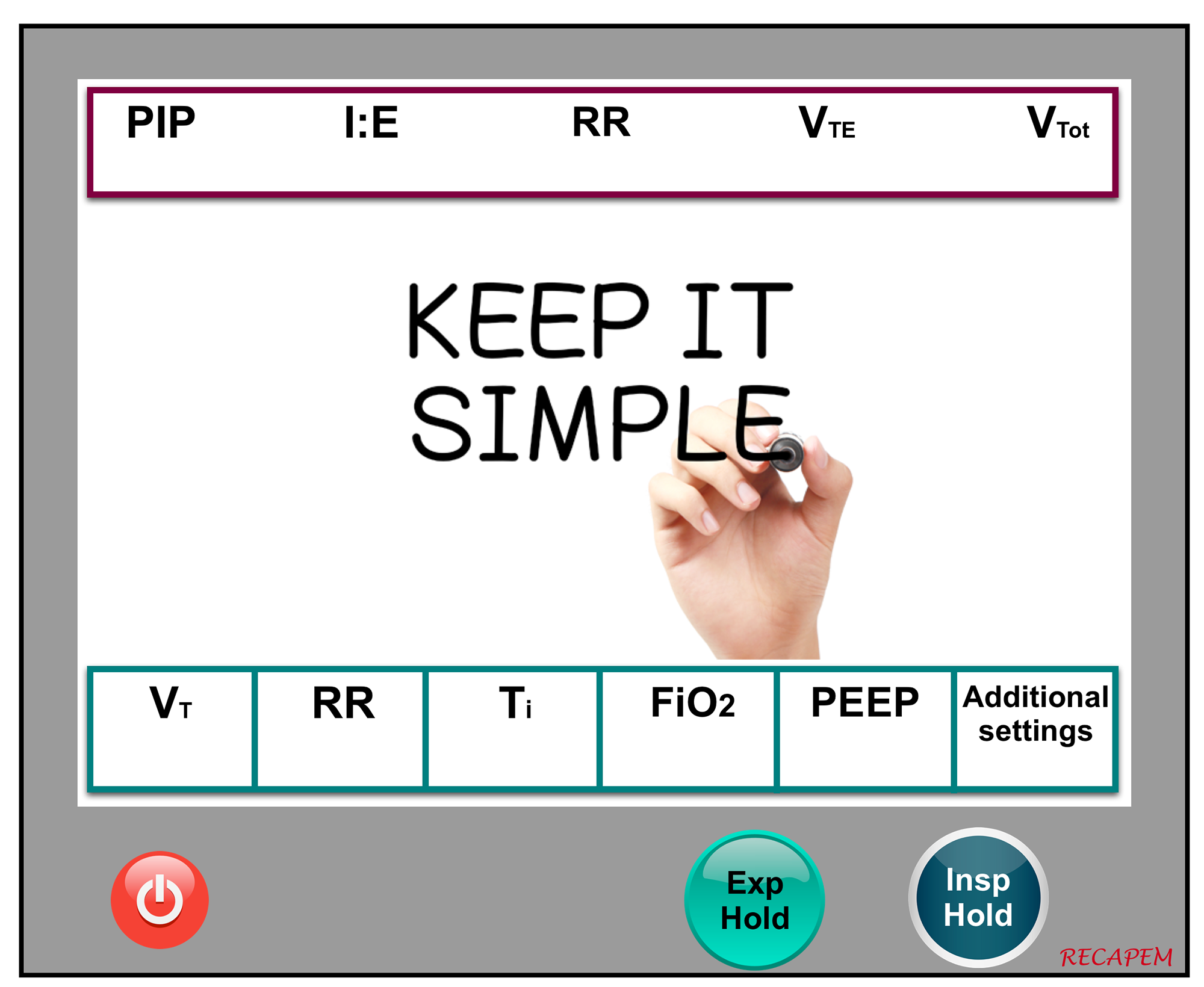
CONTENTS
- Preface
- Respiratory failure
- Introduction to MV
- Physiology of Positive pressure ventilation
- Foundational concepts
- Ventilator induced lung injury (VILI)
- Management strategies
- Ventilator alarms
- Approach to crashing patients on the ventilator
- Approach to hypoxemia & ventilator alarm
- RECAP
- Media
- Going further
- References
Preface
The purpose of mechanical ventilation is to support oxygenation and ventilation without further inducing lung damage; that is to say sending lungs to vacation and having them rest for a while. In this pictorial post, this fundamental concept is explored! If you take nothing else from this post, you should at least follow the principles that are summarized here.
Acute Respiratory failure
In the previous post (here) the pathophysiology and definition of respiratory failure was explored. For the purpose of this conversation, it is worth mentioning how we can fix respiratory failure by mechanical ventilator. From this perspective let’s classify respiratory failure broadly into 3 categories:
- ARF due to primary lung problems such as pneumonia, ARDS etc.
- ARF due to secondary lung problems such as iatrogenic pulmonary edema due to volume overload.
- ARF due to non-pulmonary causes such as diabetic ketoacidosis etc.
Obviously ventilator does not fix non-pulmonary causes of ARF. Moreover it may not fix certain pulmonary conditions either.
In the context of mechanical ventilation, it seems wise to envision respiratory failure as two big problems.
- Ventilation problem (figure 1).
- The measure for arterial oxygenation is (PaO2: FiO2)
- <300: Mild hypoxemia.
- 200-300: Moderate hypoxemia.
- <100: Severe hypoxemia.
- The measure for arterial oxygenation is (PaO2: FiO2)
- Oxygenation problem (figure 2).
- The measure for ventilation is PaCO2 which is obtained from ABG (or VBG).
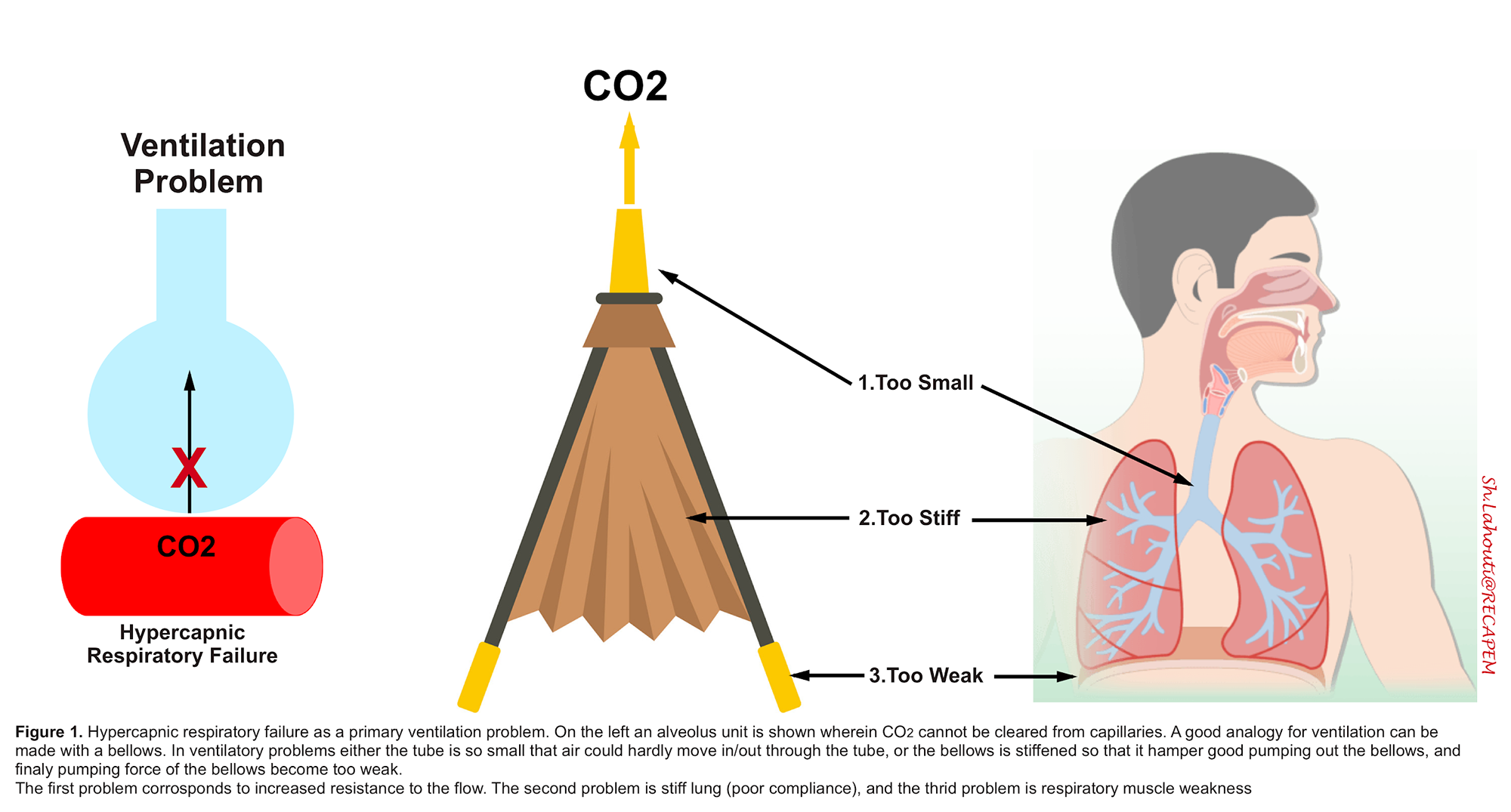
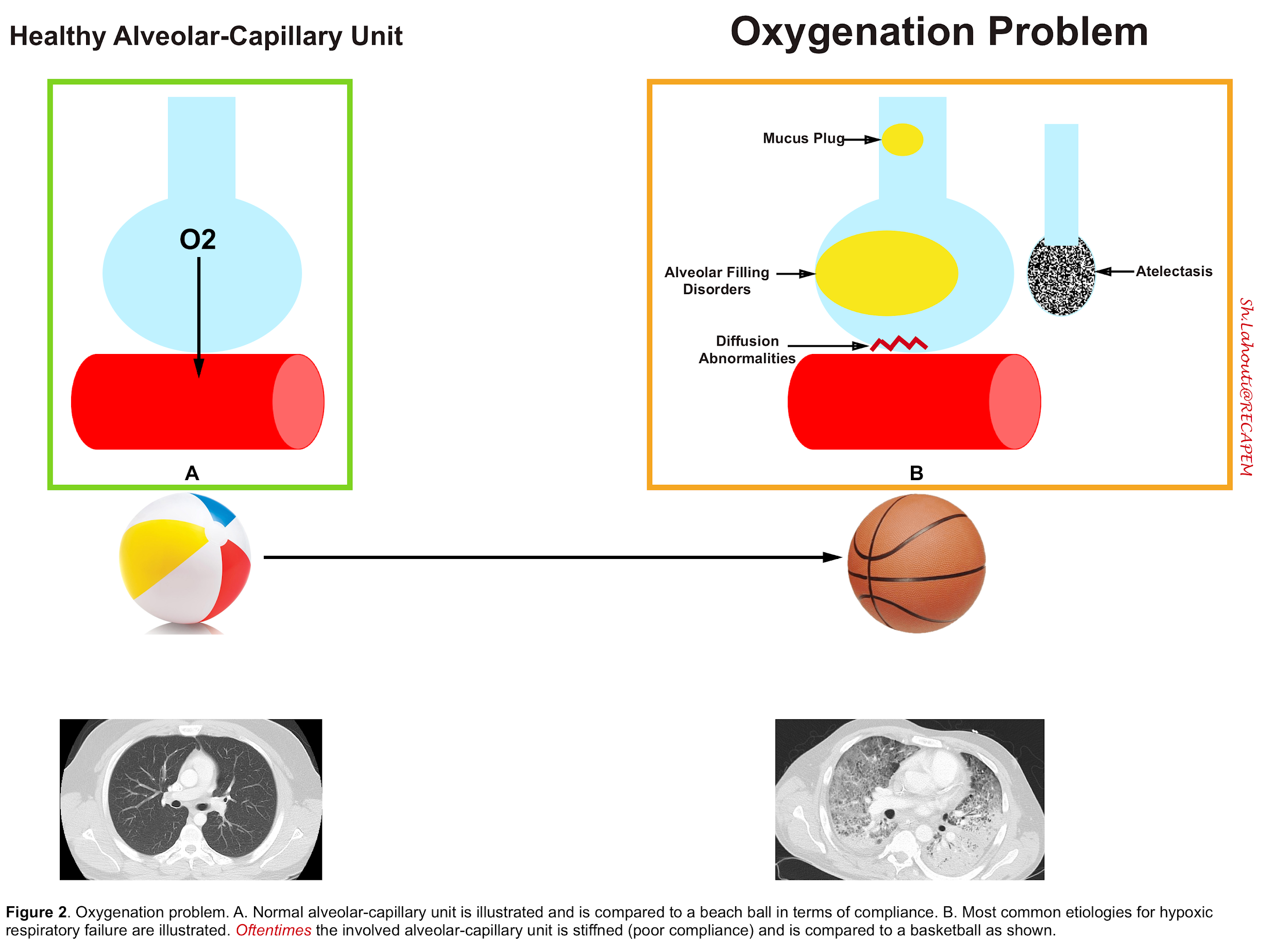
Patients with oxygenation problems “often” have a non-compliant lung (stiff lung compared to basketball) and therefore they suffer from both oxygenation and ventilation insufficiency (Hypoxic and Hypercapnic Respiratory failure). On the other hand, patients with ventilation problems often have too small or too weak bellows.
Introduction to mechanical ventilator
The purpose of the following discussion is to define essential parameters and how these are mapped out in the ventilator (figure 3). As illustrated below, the lower bar shows the different parameters that you can set for your patient (what you program to happen). The upper bar shows what is actually happening and the middle bar shows the waveform which gives you a hint for the cause of what is happening to your patient.
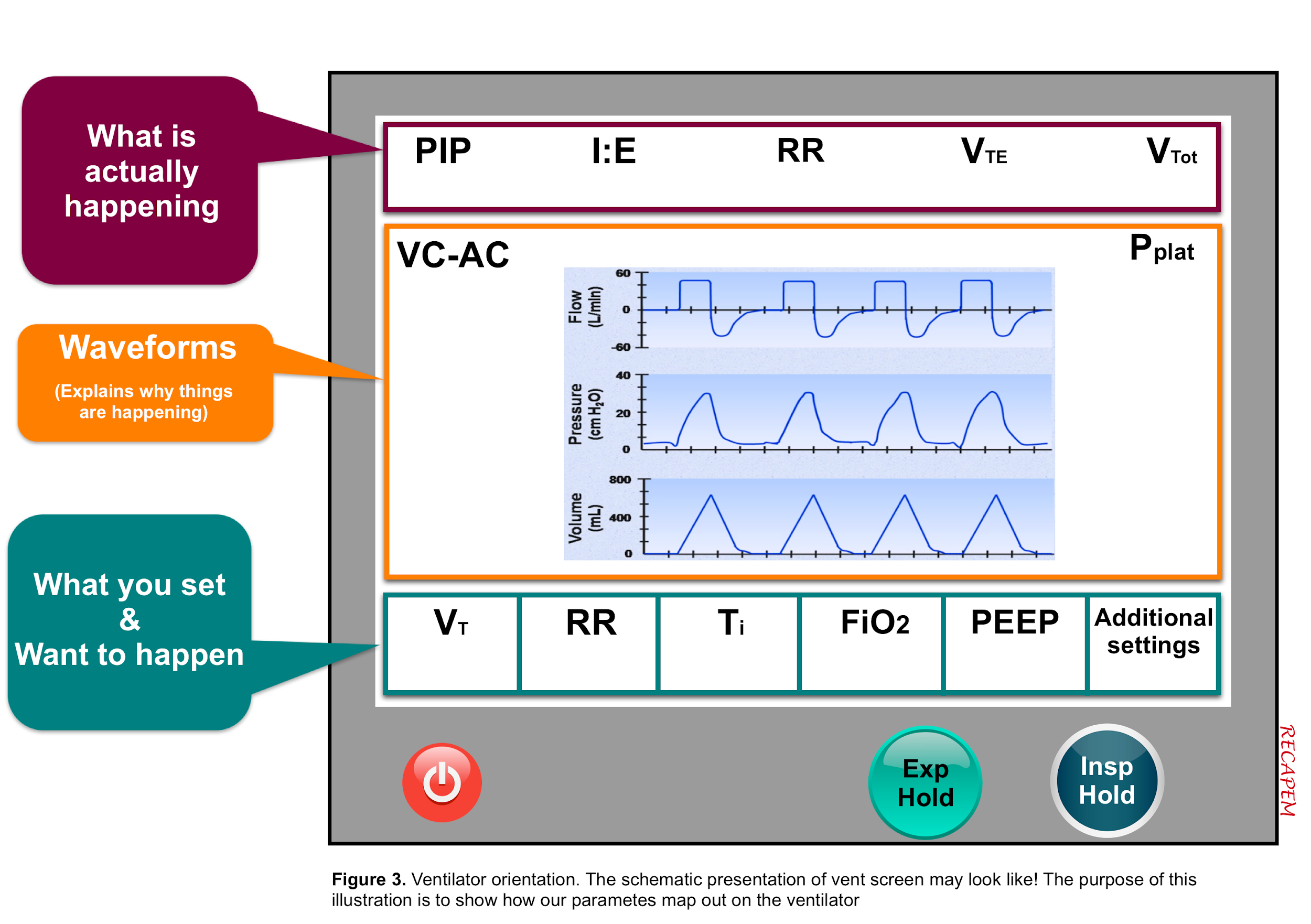
Some basic definitions 1
- Tidal volume (VT): The volume of gas delivered during each breathing cycle.
- Minute ventilation = respiratory rate × tidal volume
- PEEP: Airway pressure maintained during the expiratory phase
- Ti: Inspiratory time. The time that is spent per each breath for inspiration
- VTE (on the upper bar): Is the tidal volume that is actually being delivered
- Vtot (on the upper bar): is the minute ventilation that is actually being delivered
- I:E ratio: Is the ratio of time spent in inspiration relative to expiration
There are 3 basic physiologic concepts which are essential and you should digest it; otherwise the whole discussion looks alien to you!
- Pressure-volume relationship
- P = V/C (whereas P = pressure, V = volume, C = compliance).
- This implies that in a given system volume and pressure are interrelated (if you increase the volume, the pressure will rise and vise versa).
- Not surprisingly, if you increase the tidal volume, the airway pressures will rise for a given lung compliance.
- Compliance
- Lung compliance is a measure of the lung’s ability to expand.
- Low compliance indicates a stiff lung and can be thought of as a basketball
, whereas high compliance indicates a pliable lung and can be thought of as a balloon
(figure 4).
- Within a closed system, a given amount of volume creates a certain amount of pressure for that system. The relation between the change in volume (ΔV) to change in pressure (ΔP) determines how compliant your system is.
Compliance = ΔV: ΔP
- Airway Resistance
- Flow is proportional to the radius to the power of four.
- If the diameter of the airway is decreased by even tiny amount, the change in flow would be substantial (figure 5)
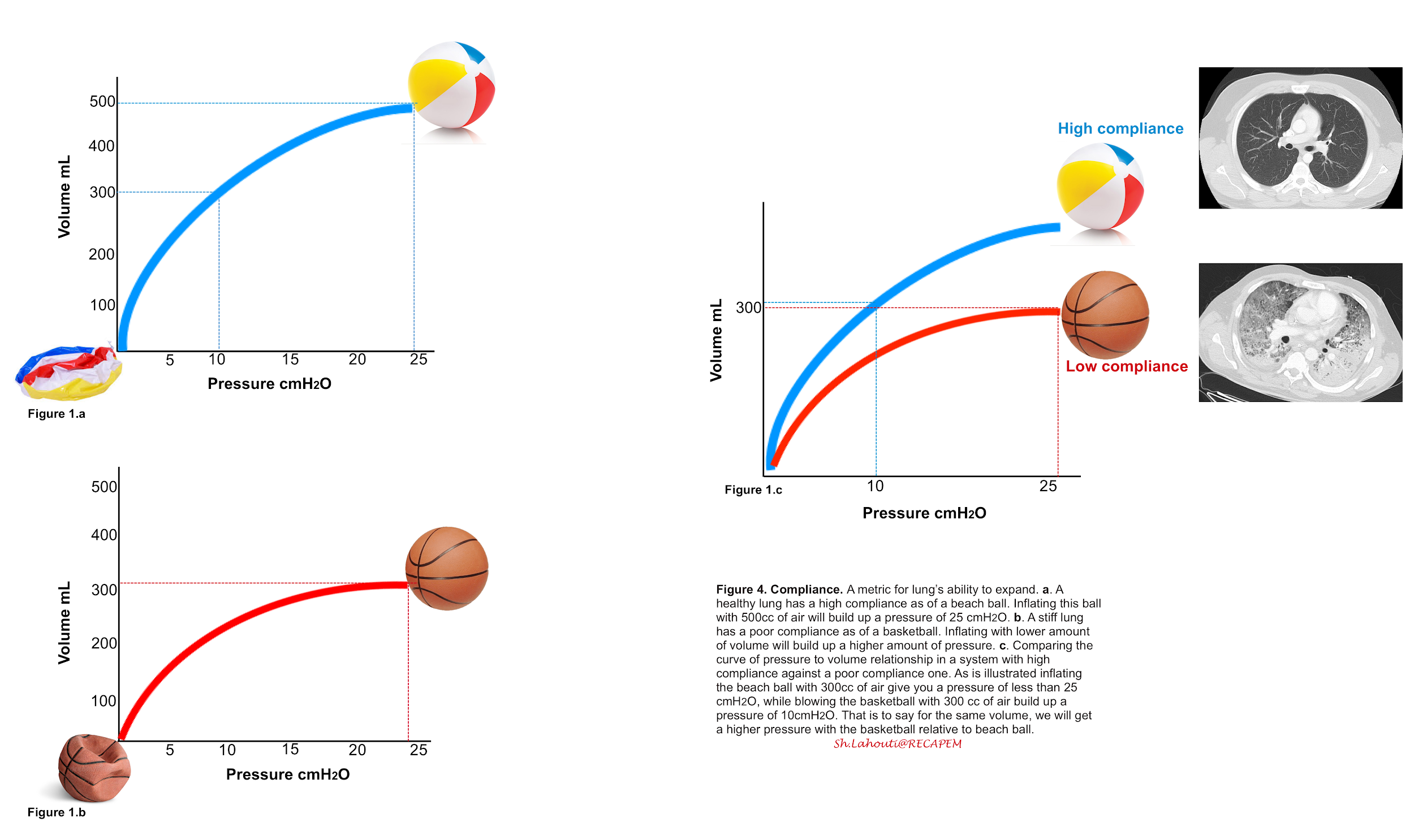
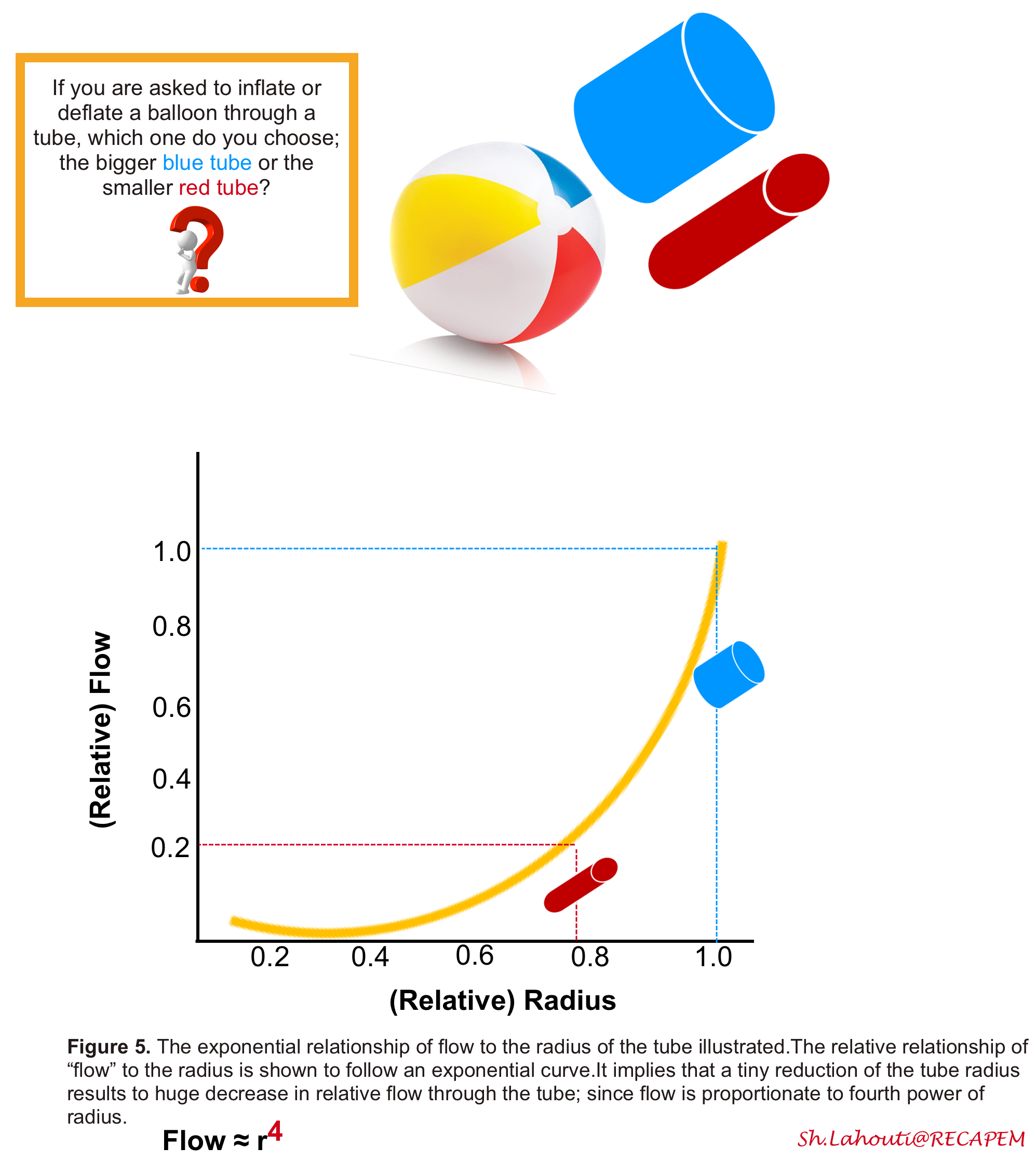
Physiology of positive pressure ventilation
Normal respiration is a process of negative pressure ventilation in which the respiratory muscles and diaphragm contract to create negative pressure in the thorax. This negative pressure allows air to flow into the airway and alveoli.
Mechanical ventilation reverses the physiology and creates a positive pressure that drives air into the lungs. If it’s about the pressure, let’s briefly discuss the pressures in the respiratory tract! A schematic airway pressure is shown in the following figure during mechanical ventilation (figure 6).
- PEEP
- PEEP is the airway pressure maintained during the expiratory phase. It is generally added to mitigate end-expiratory alveolar collapse.
- Peak airway pressure (aka Peak inspiratory pressure; PIP)
- This is the highest pressure at any point in the system (from trachea down to alveoli) as shown below (figure 7,8). It is a parameter that you can set in a ventilator as will be discussed later in this post.
- Plateau pressure (Pplat)
- It is the pressure that alveoli are exposed to (figure 7,8).
- This is the pressure that you cannot “DIRECTLY” set in a ventilator.
- In order to figure out the plateau pressure you should press the “inspiratory hold button” and hold it for 0.5 sec, and then the ventilator will give you the plateau pressure somewhere on its screen (depending on vent model) see figure 3.
👉It is crucially important to know that PIP is totally different from plateau pressure as illustrated in figure 7. Plateau pressure is the pressure that alveoli are exposed to. A high plateau pressure may signify a non-compliant lung (e.g. ARDS). More on this below.
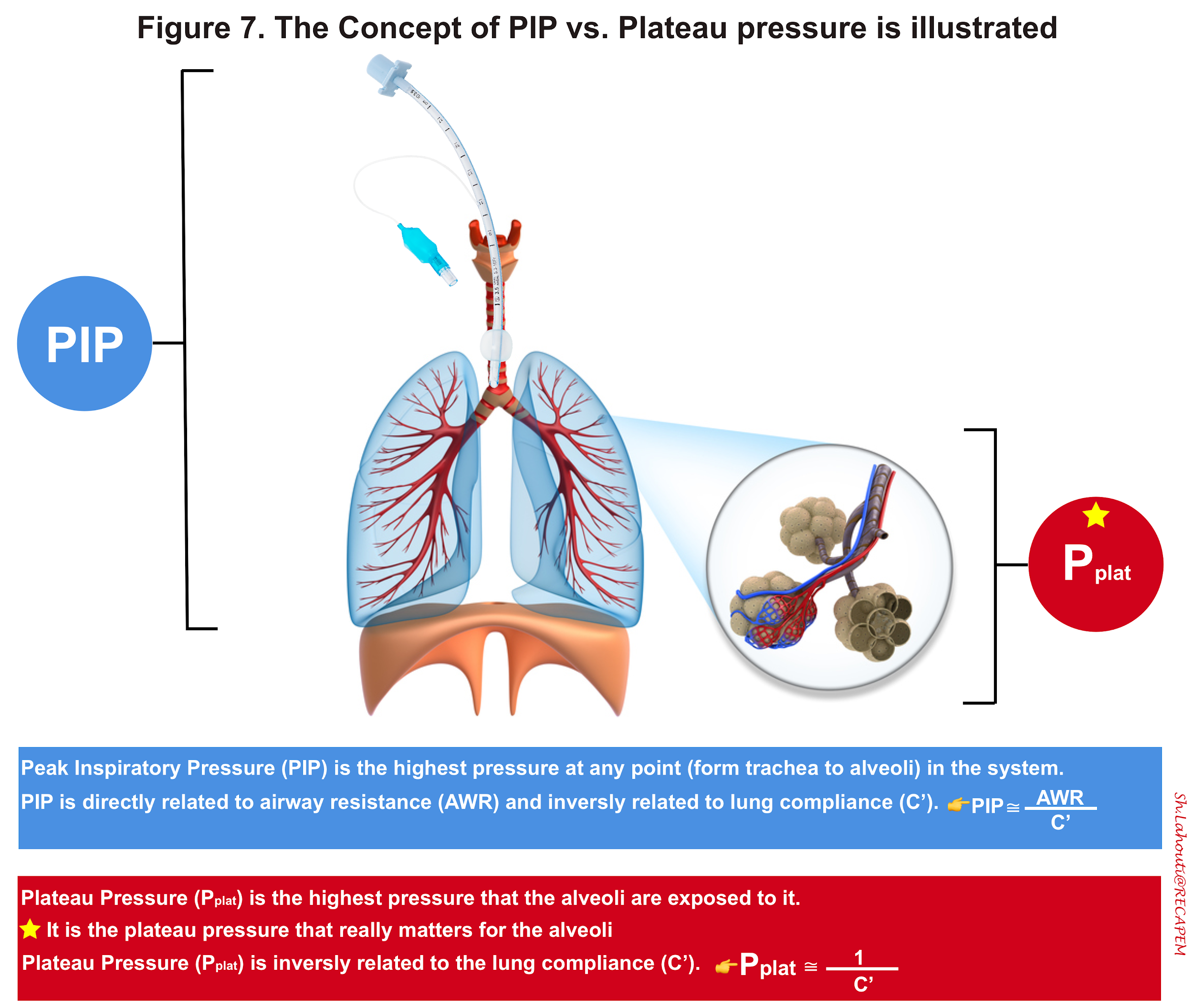
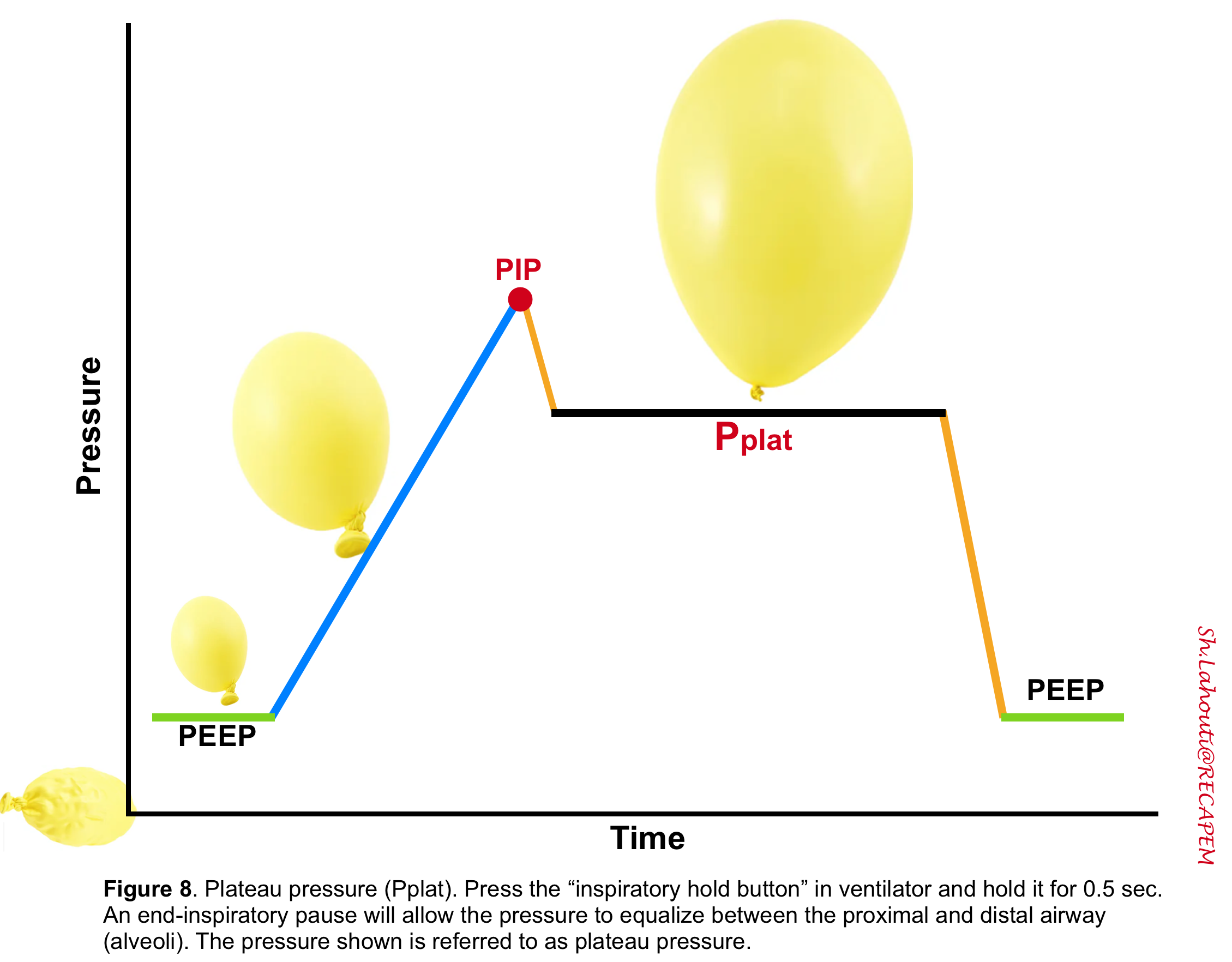
- Auto-PEEP
- It is the positive end-expiratory pressure caused by the progressive accumulation of air (air trapping), due to incomplete expiration prior to the initiation of the next breath (figure 9).
- This occurs when expiration is limited by airway narrowing or obstruction, or when expiratory time is limited.
- Driving Pressure (ΔP)
- This is the pressure above the PEEP applied to the entire respiratory system to deliver a tidal breath 2 (figure 10).
- If you inspect more (figure 10), you will easily find that driving pressure represents the pressure used to distend the chest wall plus lungs.
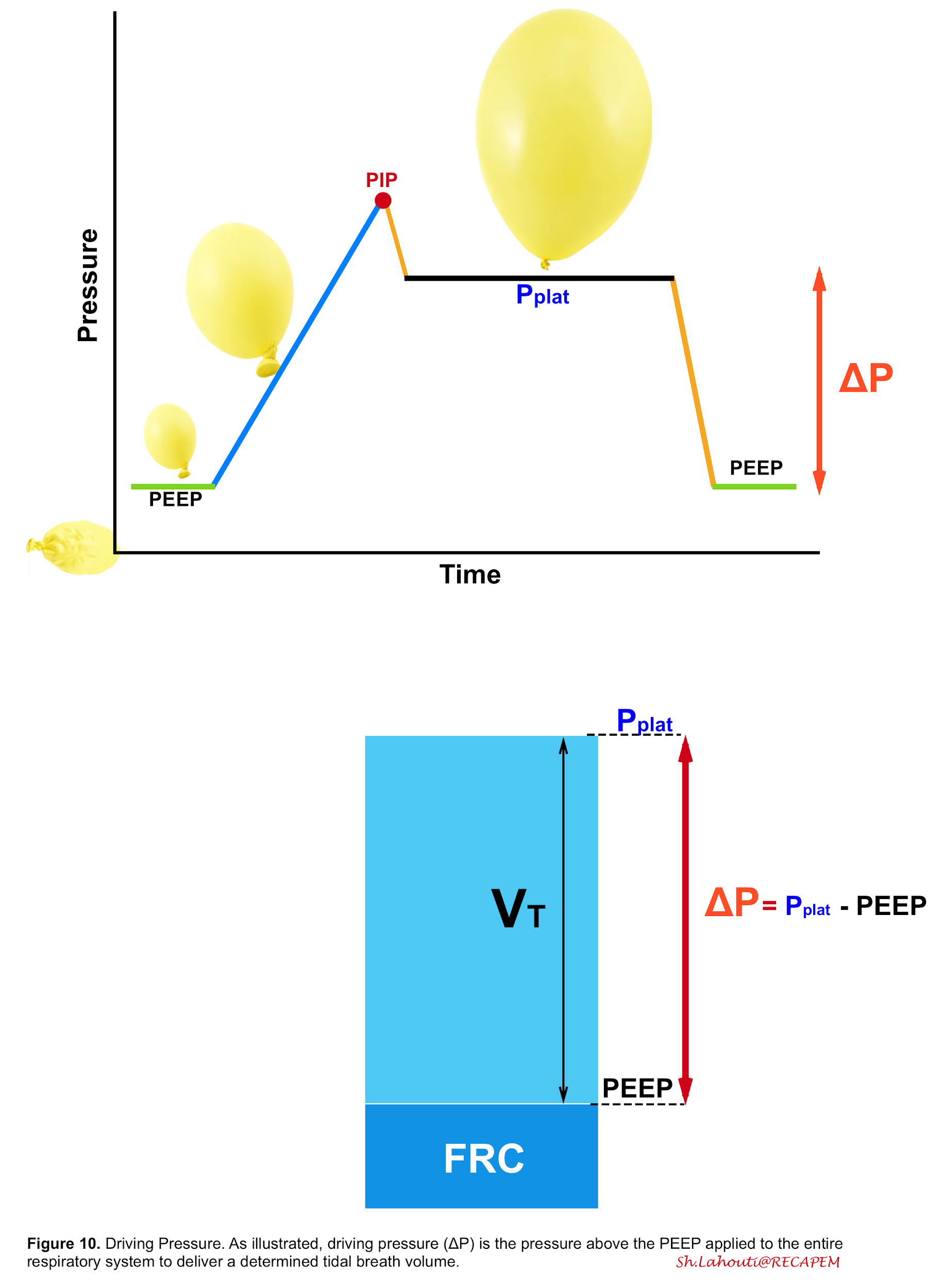
Why plateau pressure is important?
- Well, it is a crucial parameter since it represents alveolar pressure. If it is increased, you can conclude that you have a stiff lung (basketball). Right?
- To answer this question, let’s compare two cases: Case 1, a patient with ARDS whose plateau pressure is measured high; and Case 2 an obese patient with ascites who turns out to have a high plateau pressure despite a healthy lungs. How do you explain the high plateau pressure in the second case?
- Well, the respiratory system includes the lung and the chest wall, and the airway pressure (including plateau pressure) is related to the both. Therefore you may have a compliant lungs but non-compliant thoraco-abdominal compartment (e.g. obesity, ascites). In this condition, plateau pressure may mislead you regarding lung texture.
- To solve this problem, let’s get back to driving pressure and rephrase it in figure 11.
- To answer this question, let’s compare two cases: Case 1, a patient with ARDS whose plateau pressure is measured high; and Case 2 an obese patient with ascites who turns out to have a high plateau pressure despite a healthy lungs. How do you explain the high plateau pressure in the second case?
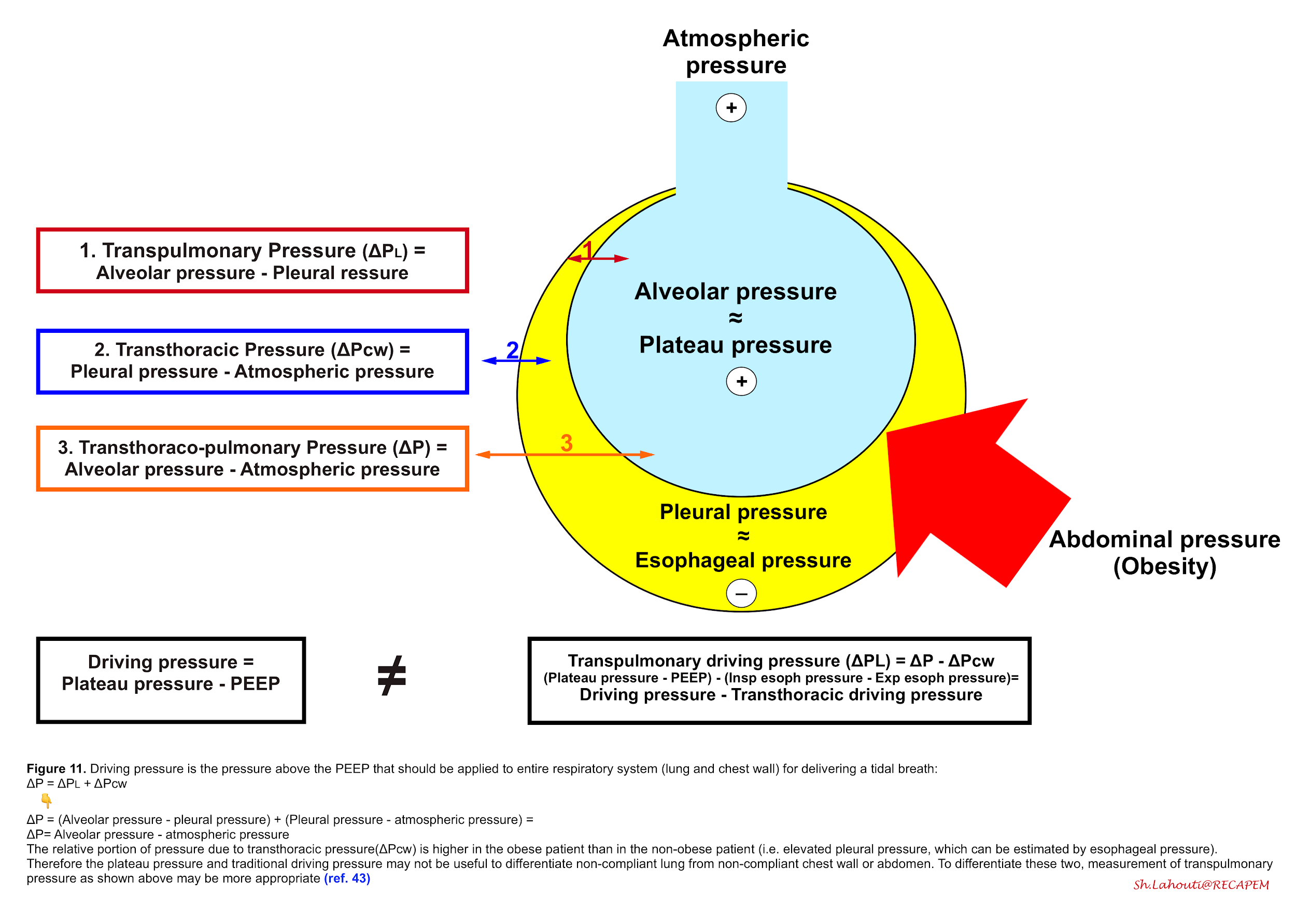
- In essence Driving Pressure (ΔP) has two components:
-
- Trans pulmonary pressure (ΔPL)
- Is the part of this driving pressure which is distributed to the lung itself.
- It is the pressure difference between the alveolar pressure and the intrapleural pressure.
- Therefore it is the mechanical load that is applied to the lung during positive pressure ventilation and is a marker of lung parenchymal stress.
- Trans thoracic pressure (ΔPcw)
- Is part of the driving pressure which is applied to the chest wall.
- Trans pulmonary pressure (ΔPL)
- From this perspective the Driving Pressure is the sum of the above two pressures (ΔP = ΔPL + ΔPcw).
- In obese patients, elevated plateau pressure may be related to an elevated transthoracic pressure, rather than an increase in transpulmonary pressure with accompanying lung overdistension 3.
- To differentiate the chest wall pressure from the lung pressure, assessing trans-pulmonary pressure using esophageal pressure (which represents intrapleural pressure) may be appropriate in patients with such non-compliant thoraco-abdominal compartment (e.g. morbid obesity, pleural effusion, massive ascites, abdominal compartment syndrome).
Basic ventilator parameter
The basic ventilator parameters which you can set (depending on the ventilator mode) are shown below (figure 12). Basic ventilator parameters are divided physiologically to those which have a prominent effect on ventilation (PaCO2) vs. those with major influence on oxygenation (PaO2).
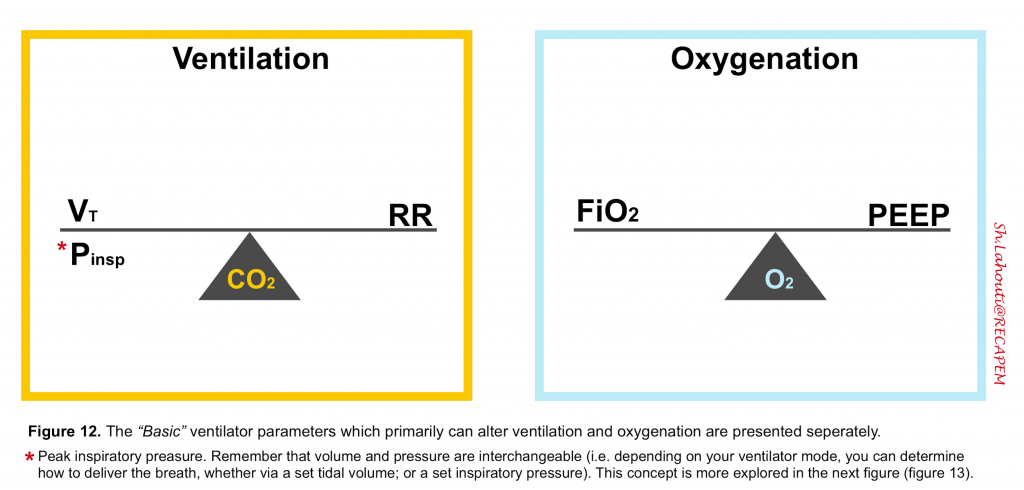
VENTILATION BASIC PARAMETERS
Tidal volume (VT)
- Is the volume of air that moves in/out during each breath. However this simple definition (in spontaneously breathing patients) becomes tricky in mechanically ventilated patients.Why?
- The answer relies on the fact that volume and pressure are interrelated (V = P × C).
- As shown in the following illustration (figure 13), there are two ways to blow off a beach ball. Either you can give a certain amount of volume, or you can give a certain amount of air at a specific pressure enough to blow up your ball. Having said that, the volume and pressure are interchangeable.
- Volume (VT): If you choose to blow off your ball with a determined volume of air, then volume is your independent variable (you directly dial it), and pressure is your dependent variable (figure 13.A).
- Inspiratory pressure (Pinsp): If you choose to blow off your ball with a determined (i.e. set) pressure, then pressure is your independent variable (you can dial it), and volume is your dependent variable (figure 13.B).
- As shown in the following illustration (figure 13), there are two ways to blow off a beach ball. Either you can give a certain amount of volume, or you can give a certain amount of air at a specific pressure enough to blow up your ball. Having said that, the volume and pressure are interchangeable.
- The answer relies on the fact that volume and pressure are interrelated (V = P × C).
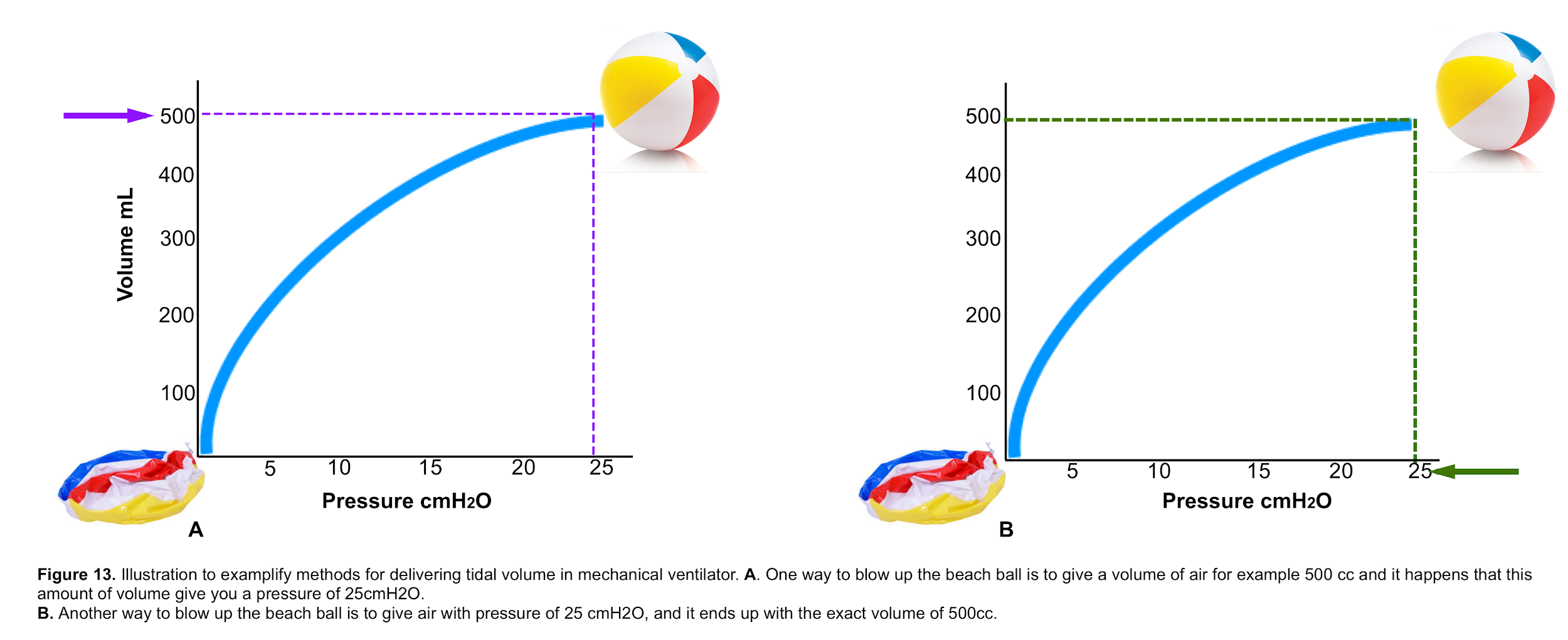
Respiratory rate (RR)
Well! On paper it makes sense that increasing the ‘RR’ can increase minute ventilation (VE= RR x VT), however it’s not really practical in mechanically ventilated patients! Why?
- Dead space
- Remember that with every breath, there’s a fixed percentage of anatomic dead space, which does not meet alveoli.
- In intubated patients this anatomic dead space is extended more (by the endotracheal tube and circuit) hence called “mechanical dead space”. Even if you ignore the latter issue, by increasing RR, there’s a cumulative effect on anatomical dead space (figure 14 below).
- Breath stacking
- Increasing the RR has another physiological influence called “breath stacking”.
- Take a breath, and before you fully exhale this breath, take another deep breath. After 3 to 4 cycles, you will find that you’re annoyed because your lungs are stacked by air and you can no longer take another breath. This is called breath stacking, which if ignored, works like tension pneumothorax physiologically (figure 29).
- Increasing the RR has another physiological influence called “breath stacking”.
Inspiration to Expiration (I:E) Ratio
- The amount of time that is spent in inspiration vs. expiration (figure 15) and in healthy spontaneously breathing individuals, the I:E ratio is about two.
- In mechanical ventilators this ratio can be set to a certain number indirectly by adjusting inspiratory time (TI) and RR.
- For example if you set RR for 10 breath per minute and inspiratory time for 1.5 seconds, then expiratory time comes into 4.5 seconds, and your I:E ratio is 1:3.
OXYGENATION BASIC PARAMETERS
👉Keep in mind that both PEEP and FiO2 can be directly set for all ventilator modes.
Fraction of inspired oxygen (FiO2)
- Generally oxygenation goals should be individualized and hyperoxia should be avoided. For most patients, the lowest possible FiO2 necessary to meet oxygenation goals should be used, ideally targeting a SaO2 between 90 and 96 percent, if feasible 4.
- 👉Remember that in patients with alveolar filling disorders such as ARDS, titrating up the FiO2 is not helpful since the inspired O2 does not meet alveolar capillary unit for exchange. In such conditions titrating up the PEEP is “often” useful in improving oxygenation (will be discussed separately).
- For patients with hypoxemic respiratory failure who are difficult to oxygenate on high FiO2, or patients with hypercapnic hypoxemic respiratory failure, the limit of acceptable oxygenation may be lower. For example, a SaO2 of 88% may be acceptable in patients with hypercapnia from COPD.
Positive end-expiratory pressure (PEEP)
- Physiology (media below)
- PEEP works as splinting the alveoli open during expiration and mitigate end-expiratory alveolar collapse (figure 16.a).
- In patients with lung injury (e.g. ARDS), the alveoli are more prone to collapse and PEEP will recruit these lung units and improve oxygenation. Schematic illustration for best PEEP is presented in figure 16.b.
- Compare a fully deflated balloon with partially deflated one (figure 10). If you were asked to blow off one of these balloons, which one would you choose?
- Correct! The partially deflated balloon takes less effort to get blown off. The same is true in alveoli that are kept partially open by PEEP at end-expiration. A lower amount of pressure will be required to inflate these alveoli during inspiration, which is good!
- PEEP works as splinting the alveoli open during expiration and mitigate end-expiratory alveolar collapse (figure 16.a).
- Beneficial effects
- Prevent atelectrauma
- Frequent opening and closure of alveoli are associated with atelectrauma (more on this below). Applied PEEP will prevent atelectrauma.
- Preventing ventilator associated pneumonia
- PEEP has been shown to be associated with reduction in incidence of ventilator-associated pneumonia as it decreases the leakage of posterior pharyngeal secretions into the lower airway 5.
- Prevent atelectrauma
- Titration
- A typical initial applied PEEP is 3-5 cm H2O.
- However, up to 24 cm H2O may be used in patients undergoing low tidal volume ventilation for ARDS.
- A suggested adjustment strategy in patients with ARDS is discussed later in this post.
Basic ventilator modes
In simple words, think ventilator mode as a system that molds your basic ventilator parameters into specific fashion. From this perspective, you can map out your basic ventilator parameters in a fashion that the ventilator mode provides for you.You may have read this topic from several resources however in the following discussion, I will describe the basic ventilator modes 6 in a simple practical way so that you will hopefully understand and employ this concept in your practice!
What factors determine the ventilator mode? The answer to this question relies on the following two questions:
- Question 1: Is volume or pressure the independent variable?
- Modes where volume is your independent variable (i.e you set the tidal volume) are called “Volume Control Mode” (VC mode).
- Modes where pressure is your independent variable (i.e you set the inspiratory pressure) are called “Pressure Control Mode” (PC mode).
- Question 2: How does the ventilator interact with the patient? (figure 17)
- If the ventilator is driving; it’s called assist control (AC mode). You can set the RR.
- If the patient is driving; it’s called pressure support (PS mode). You cannot set the RR, as patient breath at his own rate.
- If patient is allowed to drive until he screws up and then the ventilator will take over the drive, it’s called synchronized intermittent mandatory ventilation (SIMV mode). You can set the RR.
Having these questions in back of your mind, now let’s visit some commonly used ventilator modes.
VC-AC
- Q1: The independent variable is volume (it’s a volume control mode) and therefore you will set the VT in the ventilator.
- Q2: Ventilator is driving, so you will also set the RR in the ventilator.
PC-AC
- Q1: The independent variable is pressure (it’s a pressure control mode) and therefore you will set the Pinsp in the ventilator.
- Q2: Ventilator is driving, so you will also set the RR in the ventilator.
Pressure Support (PS)
- Q1: The independent variable is pressure (it’s a pressure control mode) and therefore you will set the Pinsp in the ventilator. However in terms of terminology, the Pinsp is called pressure support (PS) though both are the same things!
- Q2: The patient is driving, so you do not set the RR. Patient breath at his own respiratory rate.
VC-SIMV
- Q1: The independent variable is volume (it’s a volume control mode) and therefore you will set the VT in the ventilator.
- Q2: Patient is driving unless he screwed up and the ventilator takes over and drives then. Therefore you will also set the RR in the ventilator.
However here you will set another parameter as well and that is Pressure Support (PS):
- If the patient breathes at his own rate, then the ventilator is OK with that and just gives a pressure support that you have already set.
- If the patient stops breathing, the ventilator senses and starts delivering the breaths at the tidal volume that you have set (Vent is driving the patient).
PC-SIMV
- Q1: The independent variable is pressure (it’s a pressure control mode) and therefore you will set the Pinsp in the ventilator.
- Q2: Patient is driving unless he screwed up and the ventilator takes over and drives then. Therefore you will also set the RR in the ventilator.
However here you will set another parameter as well and that is Pressure Support (PS)
- If the patient breathes at his own rate, then the ventilator is OK with that and just gives a pressure support that you have already set.
- If the patient stops breathing, the ventilator senses and starts delivering the breaths at the Pinsp that you have set (Vent is driving the patient).
Other ventilator variables
Now that we have made our mental framework for ventilator modes, let’s talk briefly about other parameters that you are allowed to directly adjust for each specific mode.
Variable related to Q1 (above); when your mode is set for volume control
- Peak Flow Rate
- It refers to how fast or slow you can deliver your inspiratory flow. You can set this variable for volume control modes directly. Adjusting the flow rate to appropriate range will bring comfort for the patient. Let’s see how it works!
- If you choose the RR of 16 and I:E ratio of 1:2 or 1:3 for your patient in a volume control mode, a flow rate of 40-60 L/min is often reasonable.
- In contrast, in a patient with obstructive physiology you often need to adjust your I:E ratio to lower points like 1:4. In such conditions you’re functionally limiting inspiratory time (Ti) and extending the expiratory time. In order to deliver the same amount of volume during a shorter inspiratory time, you need to increase the flow rate.
Let’s get back to our nice beach ball
. If you were asked to blow off your ball faster in a shorter period of time, obviously you should blow off faster, isn’t it! Here you’re inflating your ball at a higher flow rate.
- What is the upper limit of flow rate which you can dial on the ventilator?
- Peak flow rates >75 L/minute are not typically needed and may be harmful.
- However in obstruction physiology (e.g COPD) you may need to titrate up to 100L/min depending on circumstances.
- 👉Keep in mind that peak flow rate can be set just for volume control modes.With pressure control modes, the inspiratory flow rate cannot be set directly (i.e. you cannot dial a value for flow rate on ventilator).
- It refers to how fast or slow you can deliver your inspiratory flow. You can set this variable for volume control modes directly. Adjusting the flow rate to appropriate range will bring comfort for the patient. Let’s see how it works!
- Flow pattern
- Ventilators can deliver several inspiratory flow patterns including
- Square wave (constant flow)
- Ramp wave (decelerating flow)
- Sinusoidal wave(figure 18).
- Have you ever noticed the pattern of your own breathing 🤔?
- In fact, at the beginning of inspiration much air is moved into your lung and as you get closer to the end-inspiration, the amount of inhaled air is reduced. This physiologic pattern of inspiratory flow is called ramp wave (decelerating flow shown below).
- In mechanically ventilated patients the ramp wave is typically used since it may distribute ventilation more evenly than other patterns of flow, particularly when airway obstruction is present 7.
- From this perspective the pressure control modes have the advantage of providing decelerating inspiratory flow (while in volume control modes, the inspiratory flow is constant).
- Ventilators can deliver several inspiratory flow patterns including
Pressure regulated volume control (PRVC) is a smart way for delivering a breath (it’s not actually a mode). Here it takes advantage and combines the beneficial features from both volume control modes (i.e. providing a consistent tidal volume per breath) and pressure control modes (i.e. providing a decelerating inspiratory flow) to deliver a breath in a more physiologic pattern.
Variable related to Q2 (above); when your mode allows a patient to drive
When the ventilator is driving and is giving the mandatory breath (e.g. AC mode) the ventilator determines when to deliver a breath; which is set directly by you (i.e. respiratory rate).
However when a patient is driving (e.g. PS, SIMV), it is the patient that determines when a breath should be given and the ventilator works as an assistant and provides the breaths upon patient’s demand.
How does a ventilator sense this demand?
- Ventilator can sense the patient’s inspiratory effort when his efforts cause a change in the pressure or the flow in the circuit and the ventilator recognizes these changes and delivers a breath. So patients trigger a breath and ventilator senses this demand upon a change in either flow or pressure in the circuit.
Oftentimes in practice, it is preferred that the ventilator senses the flow change in the circuit (flow-triggered) relative to sensing the pressure change (pressure-triggered) 8 9.
- During flow-triggering, the trigger sensitivity is usually set at 2 L/min, which means that ventilator-assisted breaths will be triggered once the patient’s inspiratory effort generates a flow of 2 L/min.
- When pressure-triggering is used, a ventilator-delivered breath is initiated if it senses a negative airway pressure generated by the patient trying to initiate a breath that is greater than the trigger sensitivity.
- A trigger sensitivity of -1 to -3 cm H2O is typically set, which means that ventilator-assisted breaths will be triggered when the alveolar pressure decreases to 1 to 3 cm H2O below atmospheric pressure
The trigger sensitivity should allow the patient to trigger the ventilator easily. A trigger sensitivity that is too sensitive may cause a breath to be delivered in response to patient movement or subtle pressure deflections caused by water moving within the ventilator tubing. In contrast, a trigger sensitivity that is not sensitive enough increases patient effort. This may cause a prolonged period between the initial effort and the ventilator breath or patient-ventilator asynchrony.
Mechanical ventilation induced lung injury
Before a deep dive into ventilator management for specific pathophysiologies, one should grasp how mechanical ventilation may cause lung injury (ventilator induced lung injury).
- By definition, ventilator induced lung injury (VILI) is an acute lung injury affecting the airways and parenchyma that is caused by or exacerbated by mechanical ventilation.
- The principal mechanisms of “VILI” relates to Volutrauma, Barotrauma, Atelectrauma and Shear Strain 10 11.
VOLUTRAUMA (ALVEOLAR OVER-DISTENSION) |
- This is related to high tidal volume!
- Lung units are injured when faced with high tidal volume (e.g. 10cc/kg which was used in the past). In simple words, lungs hate to meet high tidal volume 12 and the resulting injury is technically called volutrauma.
- Although both high volume or a high pressure can adversely impact lung units, it has been shown in several animal studies that lungs hate “high volume” more than “high pressure” (figure 19).
- Moreover, even when you choose an apparently low number for VT (e.g. 6cc/kg), lungs still may suffer from volutrauma! This concept is referred to as baby lung 13 as illustrated in the following figure (figure 20).
- It refers to conditions where there is heterogeneous consolidation or atelectasis (eg, patient with ARDS), wherein a disproportionate volume from each breath is delivered to the open alveoli. This can cause regional alveolar overdistension and ‘VILI’ despite delivery of conventional tidal volumes that are based upon body weight (for height).
- A similar process is at play when lung compliance is reduced such as severe fibrosis or during single lung ventilation (right mainstem intubation) when a tidal breath is distributed to only one lung as opposed to the two.
- It refers to conditions where there is heterogeneous consolidation or atelectasis (eg, patient with ARDS), wherein a disproportionate volume from each breath is delivered to the open alveoli. This can cause regional alveolar overdistension and ‘VILI’ despite delivery of conventional tidal volumes that are based upon body weight (for height).
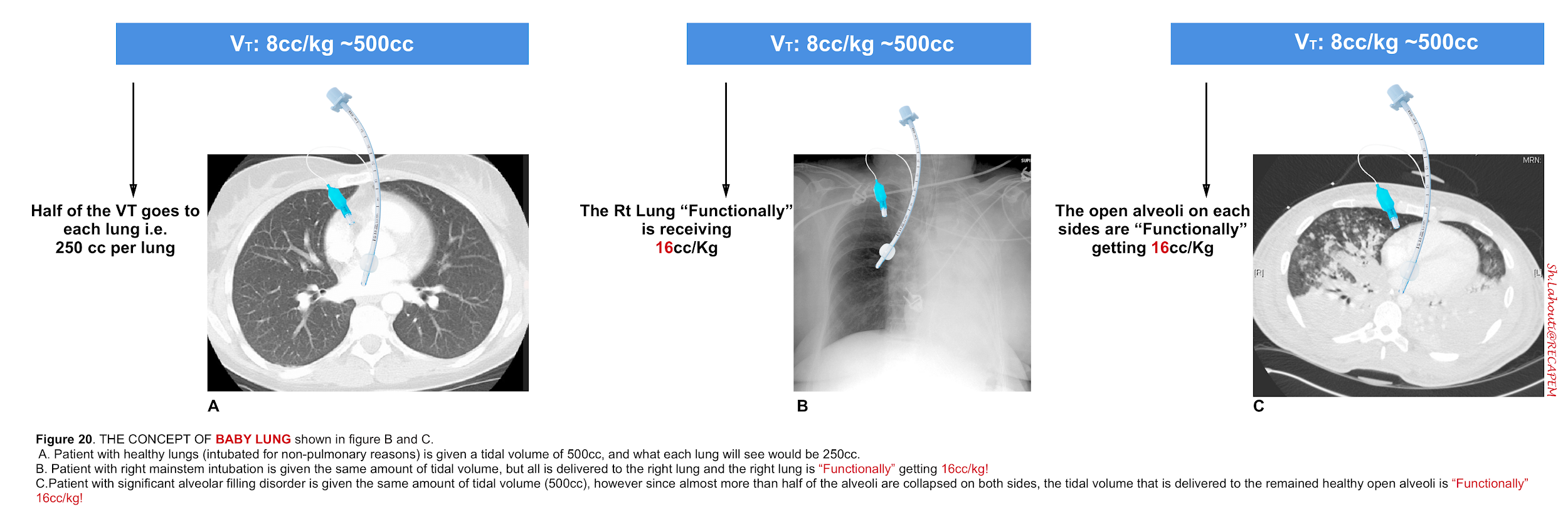
BAROTRAUMA |
- Is related to transpulmonary pressure! (See figure 10, 11).
- It refers to trauma induced by high pressures (an increased difference in pressure between alveolar pressure and its surrounding external environment, i.e. pleural pressure).
- This causes both gross barotrauma (i.e. alveolar rupture causing pneumothorax) and deleterious microscopic lung injury.
- Not only certain disease processes per se are considered as a risk factor for barotrauma (asthma, COPD, chronic interstitial lung disease, and ARDS), but also mechanical ventilation is frankly associated with it too.
- We’ve already learned that volume and pressure are interrelated, so you may say that volutrauma may cause barotrauma too. That is correct, but several studies have shown that the major risk for barotrauma is intra-alveolar pressure gradient.
- How do I monitor and prevent barotrauma?
- Pplat: The higher the Pplat, the greater the risk of barotrauma with the highest rates occurring in those with a Pplat >35 cm H2O 14.
- Transpulmonary pressure is defined as difference in pressure inside the lung vs. outside the lung. As explained before; it is a measure of lung stress 2. From this perspective, barotrauma may occur even at lower airway pressure if pleural pressure is extremely negative (eg, forceful inspiratory effort) 10.
- Does high level of PEEP impose the lungs to barotrauma?
- No, barotrauma is more pertinent to “transpulmonary pressure” (Pplat – PEEP), therefore as long as “lung protective strategy” is employed (low VT with a target plateau pressure ≤30 cm H2O) and recruitment of atelectatic lung is efficient, high PEEP does not contribute to barotrauma15.
- Pplat: The higher the Pplat, the greater the risk of barotrauma with the highest rates occurring in those with a Pplat >35 cm H2O 14.
ATELECTRAUMA |
- Is caused by high shear forces from cyclic opening and collapse of atelectatic but recruitable lung units!
- An injured alveoli (whether due to alveolar filling disorder, diffusion abnormality, etc) are particularly prone to collapse during expiration.
- The cycle of collapsing (during expiration) and popping open (during inspiration) eventually injures the thin alveolar membrane 16 17.
- This traumatic insult to the poor alveoli initiates an inflammatory cascade 18 which worsen the overall pulmonary functional unit (alveolar-capillary unit) performance.
- 👉Keep in mind that the already injured alveoli due to primary lung disease have a high tendency to collapse (atelectasis) and have a lower threshold for further damage from such huge volumetric change during each breath.
- How do I prevent atelectrauma?
- With applying PEEP, the alveolar collapse during expiration is minimized, therefore the cycle of closing and popping open is disrupted.
SHEAR STRAIN |
- Shear strain is defined as the angular deformation of an object relative to its resting conformation; for example if the resting object is square, shear strain produces a rhombus.
- Lung heterogeneity (e.g. ARDS) causes shearing strain!
- Lung injury also can be induced by shear strain from regional differences in lung mechanics.
- Adjacent alveoli share an interalveolar septum and are mechanically interdependent. When one alveolus is collapsed or fluid-filled and an adjacent alveolus is aerated, their shared interalveolar septum stretches to shift toward the nonaerated alveolus, introducing shear strain that causes injury (figure 21), with the shearing strain greatest closest to the collapsed or fluid-filled alveolus.
- Greater mechanical heterogeneity (shear strain) correlates with biomarkers of VILI and increased mortality.
- Lung heterogeneity is manifested on CT scan as regions of well-aerated lung adjacent to patchy ground-glass opacities and atelectasis10.
- How to reduce shearing strain?
-
-
- Prone positioning improves lung homogeneity to decrease shear strain and also increase aerated ARDS baby lung size (volutrauma).
- Proning improves oxygenation to the posterior lungs. It improves V/Q matching and allows the patient to have gas exchange along the posterior aspects of the lungs (figure 23).
- For the correct method of prone positioning see media below.
- Prone positioning improves lung homogeneity to decrease shear strain and also increase aerated ARDS baby lung size (volutrauma).
- Other strategies for improving lung homogeneity as well as other possible of breath delivery methods (i.e. APRV 22) will be discussed separately!
-
Bottom line |
- Alveolar injury results in high alveolar permeability, interstitial and alveolar edema, alveolar hemorrhage, hyaline membranes, loss of functional surfactant, and alveolar collapse (ie, findings similar to those observed in ARDS)23 .
- In the following table (figure 24.a) bedside monitoring indices and strategies to prevent “VILI” are summarized. For more on monitoring the driving pressure, one possible algorithm is presented in figure 24.b.
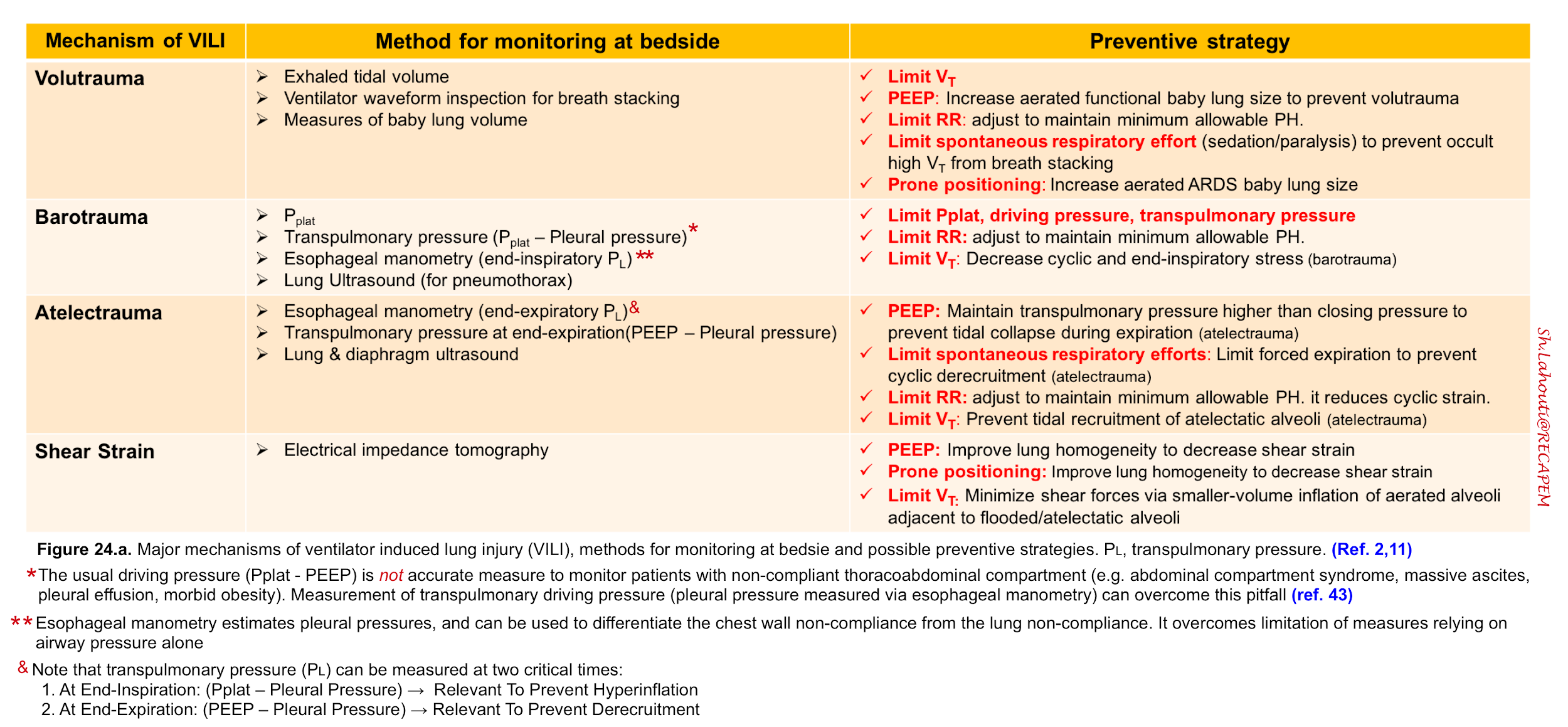
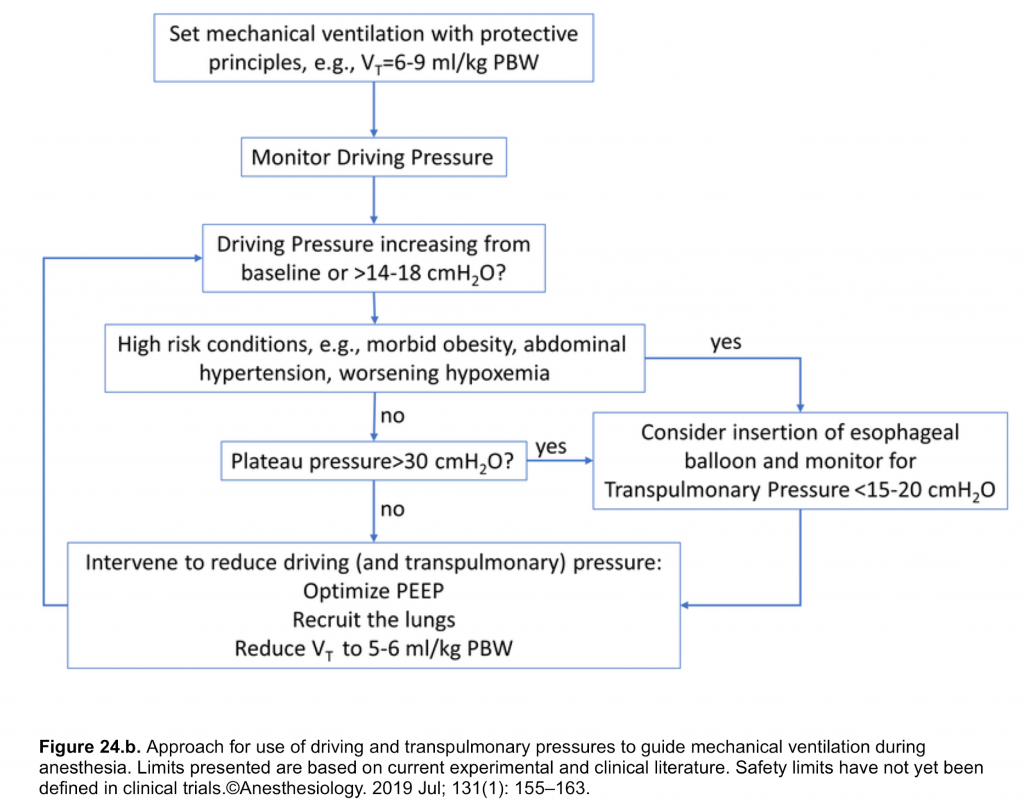
Management strategies
Despite the lack of agreement on choosing specific modes for individual patients, there is solid evidence that certain ventilatory strategies can decrease meaningful poor outcomes in ventilated patients. In the following discussion, ventilator strategies which are applied for some common specific pathophysiologies are reviewed. The common specific pathophysiologies that are discussed here are:
- Lung Injury
- Obstruction
Management Strategies for Lung Injury
This strategy is applied for patients with lung injury (e.g. ARDS). The principal is that “a Low VT protects an injured lung from further damage“!
Now that the primary mechanism of “VILI” is discussed, we can better appreciate how to employ strategies to minimize further lung damage.
The lung “Protective Strategy” is primarily referred to using Low VT (to avoid volutrauma)10 , and supraphysiologic PEEP (> 5 cm H2O) to avoid atelectrauma for an injured lung like ARDS.
How to calculate the tidal volume?
- A tidal volume is about the patient’s height but NOT weight. The lung size is related to an individual’s height but not his weight (figure 25).
- Calculate VT (figure 26) of your patients based on their height (i.e. PBW).
- In Lung injury strategy, the initial tidal volume is 6cc/kg (per height).
👉Note that the consequence of this strategy (low tidal volume) is hypercapnia (which is acceptable); hence called “permissive” hypercapnia 24 .
How to set up the ventilator for lung injury physiology? The initial setting can proceed like the following:
- Mode: AC-VC 25 or any other vent modes 10
- VT (Protection): Set at 6-8 ml/kg PBW. Reduce VT by 1 ml/kg at intervals ≤ 2 hours until VT = 6ml/kg PBW. You may even need to go further down in the presence of ARDS (see baby lung concept above).
- Further adjustment of VT is done to achieve Pplat goals (Pplat ≤ 30 cm H2O) 10 (see figure 28).
- RR (Ventilation): Initially set at 18-20. Further adjustment is based on pH and PaCO2 (Keep RR < 35bpm) 8.
- Flow rate: 60-80 L/min
- FiO2/PEEP (oxygenation): Goal is to keep PaO2 at 55-80 mmHg or SaO2 88-95% 10
- Start at FiO2 of 1 and PEEP of 5 cmH2O. Consider use of incremental FiO2/PEEP combinations.
- Despite lack of agreement on using Low PEEP vs. High PEEP scale, most clinicians proceed initially with Low PEEP scale (figure 27 the upper bar).
- A possible FiO2/PEEP titration can proceed as: Wait for five minutes and then draw an ABG. Then set the FiO2 to 40% and start titrating based on the chart (figure 27. Lower PEEP scale). Go up every 5-10 minutes; and quicker if low SaO2 .
- For patients with worsening oxygenation (PaO2:FiO2 <150) 26 despite initial measures discussed above, Higher PEEP/Lower FiO2 scale is recommended to be used 27 to recruit more alveoli and improve oxygenation (recruitment maneuver).
- The strategy that combines low tidal volume (Protection) and Higher PEEP/FiO2 scale (figure 27. Lower bar) is called “open lung ventilation” and will be discussed separately (more on this here).
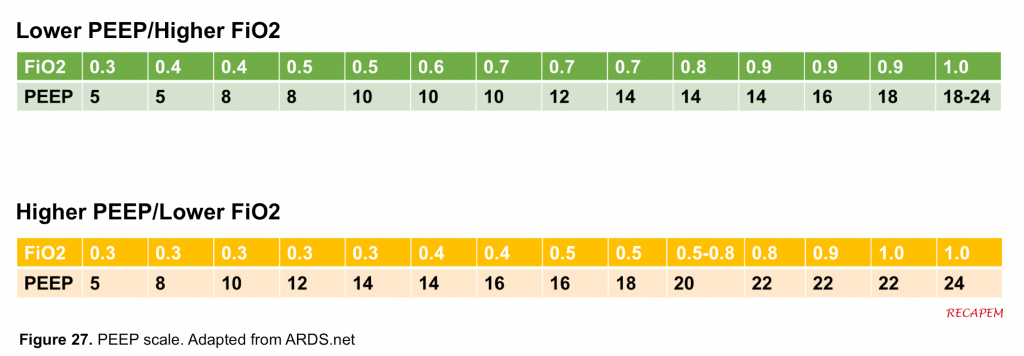
For the purpose of this discussion some points need to be explored here:
- Patients with mild-moderate oxygenation abnormalities and a more compliant lungs often respond to initial measures (low tidal volume and lower PEEP scale).
- Despite appropriate initial measures, some patients have more non-compliant lungs with poor oxygenation parameters. For these patients a higher PEEP 28 scale should be tried to recruit more alveoli and the patient’s oxygenation response be assessed 29. If appropriate response is seen, then lungs are recruitable and patient benefit from recruitment maneuvers.
- Generally the PEEP scale (figure 27) gives you a rough estimate of how to work with PEEP and FiO2 . Appropriate PEEP titration can be targeted on the following parameters:
- Oxygenation: One possible target which is most commonly used is oxygenation (PaO2:FiO2) as shown in figure 25.
- Driving pressure (Pplat -PEEP): Another possible target for PEEP titration especially for patients with severe oxygenation problems (e.g PaO2:FiO2 <150) is to monitor the driving pressure 30 31 32. This will allow clinicians to assess appropriate response of their patients to PEEP titration or other recruitment maneuvers. Although a definite cutoff value for driving pressure has not been agreed upon, several studies have shown a target value of < 20 mmHg 33 34 35. Of note monitoring driving pressure has suggested to be used for titrating tidal volume in patients with or without ARDS 36.
- Transpulmonary pressure: As already explained above, in some patients Pplat is not an accurate measure of lung texture (e.g. morbid obesity, pleural effusion, massive ascites) and therefore titrating PEEP based on driving pressure can be misleading. In these conditions “transpulmonary pressure” (airway pressure – pleural pressure) measurement can overcome this pitfall. Esophageal pressure can be measured as an estimate of pleural pressure and then transpulmonary pressure can be adjusted by titrating PEEP. Titrating PEEP to an end-expiratory transpulmonary pressure between 0 and 10 cm H2O may reduce cyclic alveolar collapse 37.
Management Strategies for Obstructive Physiology
Patients with obstructive physiology often have “primarily” ventilation problems and it usually stems from a narrowed airway (figure 1); hence the airway resistance is increased.
- This reasonably explains why intubation should be avoided as possible in patients with obstructive physiology. Such patients are already struggling to breath through a narrowed airway and now you’re going to put a tube and make their airway more narrowed (figure 5).
- If you were going to intubate these patients, use a larger endotracheal tube (e.g. ≥8 mm).
- The narrowed airway becomes more problematic during expiration, as it puts more limitations on air moving out, and eventually breath stacking develops 38.
The goal of the ventilation strategy for obstructive physiology is to give as much expiratory time as possible: lower the RR and extend the expiratory time to avoid breath stacking. The adverse effect of high RR on breath stacking is shown below (figure 29).
- By looking at the ventilator waveform (time-flow) you can figure out that your patient is breath stacking.
- Troubleshooting: Extend the expiratory time (I:E ratio of 4 or more) and lower the RR as possible.
- Keep in mind that in order to effectively extend the expiratory phase you may also need to apply a faster flow rate.
- Troubleshooting: Extend the expiratory time (I:E ratio of 4 or more) and lower the RR as possible.
- Employing lower RR often results in hypercapnia, which is allowed and acceptable (Permissive Hypercapnia) 39.
- There is no consensus regarding an acceptable lower limit for pH, which the acidosis should be corrected. However, most physicians agree that the pH should not need to be normalized and decisions regarding correction of acidosis should be individualized.
- Generally a PH of more than 7.21 to 7.24 is acceptable 40.
The possible initial ventilator setup for patients with obstructive physiology is briefed below:
- Mode: Use volume control modes initially (e.g. VC-AC, SIMV)
- Vt: 6-8 cc/kg by PBW
- I:E ratio: 1:4 to 1:5
- RR: Start at 10-12. Look for I:E ratio. Adjust the RR to achieve the set I:E ratio
- Flow rate: 80-100 L/min
- PEEP: initially zero. May need to titrate to 3-5 especially in COPD patients.
- Despite similarities between asthma and COPD, In patients with asthma the upper airway walls are stiff and solid, while in patients with COPD the airway walls are loose and collapsible. That’s why patients with COPD intuitively purse their lips!
- FiO2: Adjust to keep oxygenation. A SaO2 of >88% may be acceptable in obstructive physiology
- Permissive hypercapnia: Keep pH above 7.10.
- Do not forget sedation.
- Watch for flow-time curve on ventilator for possible breath stacking.
Ventilator Alarm
Ventilator alarm should be treated as announcing that the patient is coding (Vent alarms = CODE BLUE) because essentially until you learn why the vent is alarming, It could very well be that the patient is going to have a cardiac arrest as a result of ventilator disconnection.
Some common ventilator alarms are discussed here.
- When the ventilator is alarming that “RR is high”
- Often in this situation the patient is not well sedated.
- When the ventilator is alarming that “RR is Low”
- This often happens when a patient’s respiratory drive is trending down and is being ventilated on pressure support mode (PS mode).
- One can fix the problem by switching the mode into assist control mode (AC).
- When the ventilator is alarming that “VT is low” (in PC mode)
- Remember that in PC mode, your independent variable is “PIP” (which you set it), and VT becomes your dependent variable.
- If lung compliance is decreased (compared to basketball), then at your preset ‘“PIP”, the lower amount of tidal volume would be delivered and the ventilator alarm goes off (figure 30) 41.
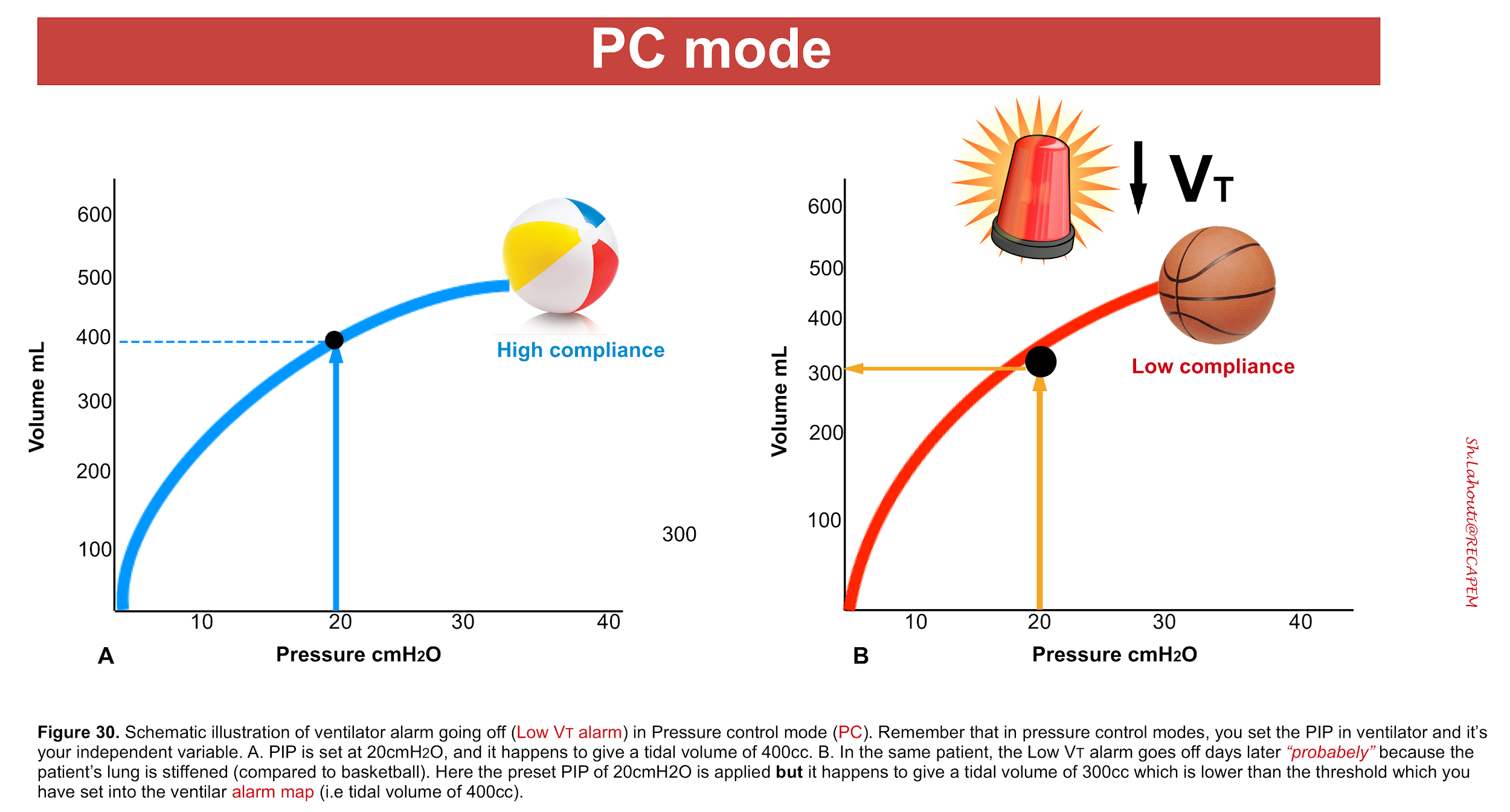
- When the ventilator is alarming that “PIP is high” (in VC mode)
- Remember that in VC mode, your independent variable is tidal volume (which you set it), and “PIP” becomes your dependent variable.
- When the ventilator is alarming “HIGH PIP”, the ventilator cuts off the tidal volume at the threshold PIP that you have set in the ventilator alarm map (figure 31).
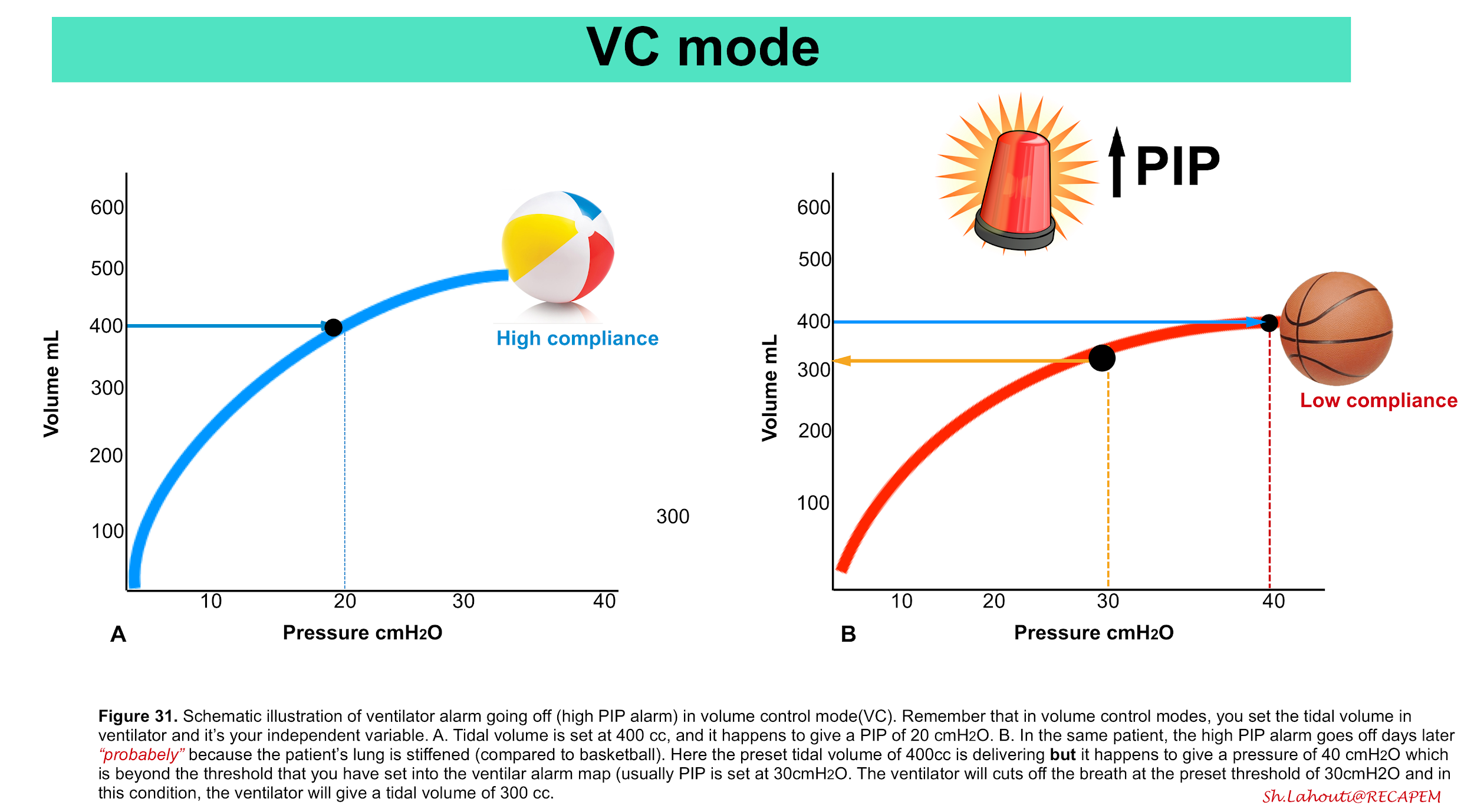
-
- Note that the ventilator alarm is based on Peak Inspiratory Pressure (PIP). However “PIP” totally differs from Plateau Pressure (Pplat) see figure 7 and 8.
- Just because PIP is high, it does not necessarily mean that Pplat is high too 41 42 ;that’s why you need to grasp the concept of PIP vs. Pplat (explained earlier)!
- The differential diagnosis of elevated both (PIP and Pplat) vs. high PIP with normal Pplat is represented below (figure 32).
- By enlarge when both are elevated, it signifies worsening lung compliance, while elevated PIP alone may happen with increased airway resistance (e.g. kinked tube, bronchospasm).
Approach to Crashing Patients on the Ventilator
If the patient is crashing on the ventilator the first action is to disconnect the patient and initiate bagging him (with PEEP valve). See the following algorithm. You will now intuitively find each step reasonable!
Clinical features of crashing patients (cardiac arrest/near arrest)
- New unexplained and rapidly dropping SBP < 80 mmHg, rapidly desaturating to SaO2<80%, extreme bradycardia or tachydysrhythmias, cardiac arrest while on mechanical ventilation.
Differential diagnosis
-
Keep in mind the “DOPES” mnemonic to rapidly consider the primary etiologies of the crashing ventilated patients 1:
-
D: Displaced tube, dysrhythmias
-
O: Obstructed tube
-
P: Pneumothorax
-
E: Equipment malfunction
- S: Stacked Breaths (Auto-PEEP).
-
Management
- Immediately disconnect from ventilator (this also allows for expiration of stacked breaths).
- Give manual bag ventilation with oxygen 100%.
- Bagging should be given by the provider but not anyone else, because this lets you get a sense of what the potential problem is (resistance to bagging).
- Pay particular attention to the delivery of manual ventilation. Limit the respiratory rate to 8 to 10 breaths/min.
- Excessive rates will increase intrathoracic pressure and lead to a decrease in venous return and cardiac output
- Look for chest rise, listen and feel for cuff leak.
-
If the patient improves when disconnected from the ventilator, it is likely an equipment (ventilator) or ventilator setting etiology.
-
If the patient has a long expiration after being disconnected from the ventilator and then stabilizes, consider auto positive end-expiratory pressure (PEEP) as the etiology (breath stacking).
-
-
If respiratory distress or hemodynamic instability persists despite disconnecting the patient from the ventilator and ventilating with 100% oxygen via a bag valve mask:
-
Evaluate for the following life-threatening conditions and treat accordingly:
-
Tension pneumothorax: Consider U/S of the chest and needle decompression
- Embolism (thrombus, air, fat): Consider bedside echo, evidence of DVT in POCUS?
-
Large pleural effusion: Perform U/S, consider therapeutic thoracocentesis.
- Pulmonary edema: U/S, echo.
-
- In the absence of life-threatening condition, assess for resistance to bagging.
- If bagging is difficult: Consider ETT obstruction and try to pass suction catheter (see the below algorithm).
- In the absence of difficult bagging, consider air leak. Evaluate by capnography and cuff pressure. May need ETT replacement.
-
Approach to hypoxemia and ventilator alarm
In situations where a patient is not crashing (no profound hypotension or significant drop in SaO2 from baseline); disconnecting the ventilator as a knee-jerk response to ventilator alarming or to mild-moderate but stable new hypoxemia may not be the best option as derecruitment happens. The mental checklist to figure out what is going wrong with your patient is illustrated below (figure 34).
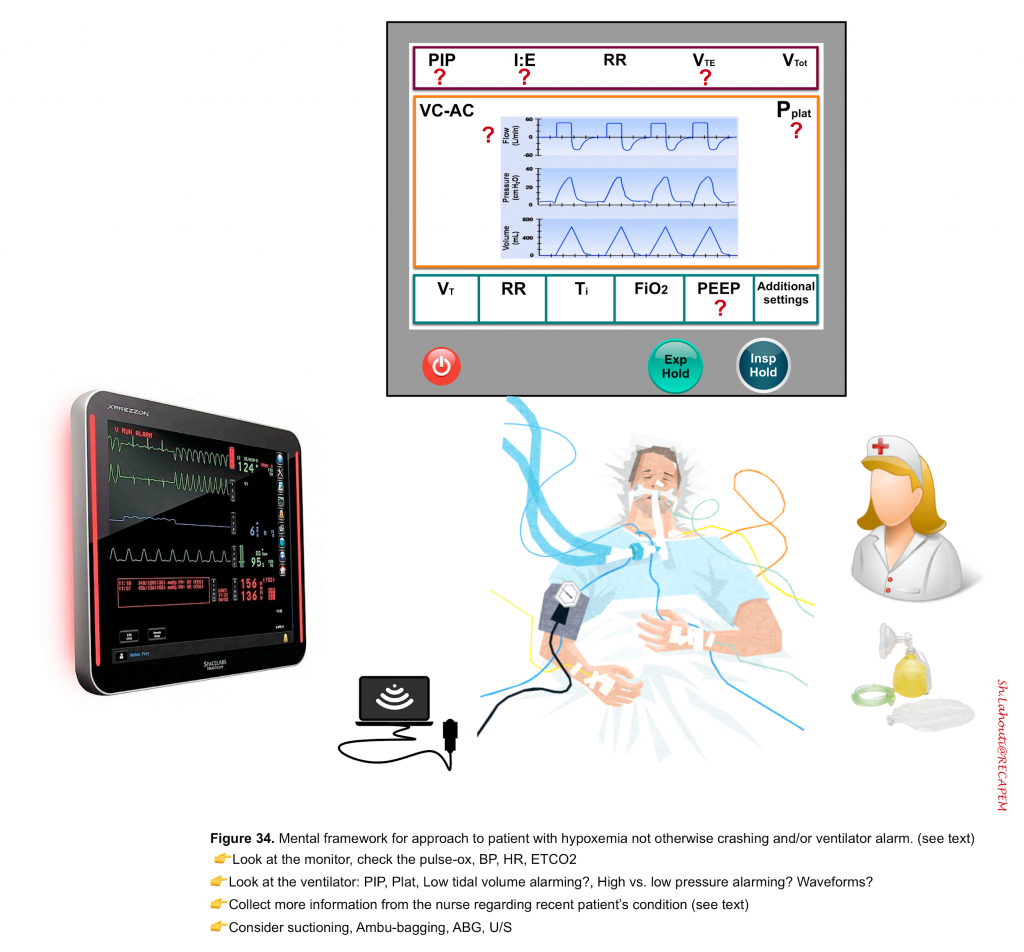
First look at the monitor
- If the pulse-ox is reading low, does the O2 waveform look good? Is BP low?
- Check blood pressure, HR?
- Does the ETCO2 waveform look good? If so the tube is not displaced!
Look at the ventilator 42
- Do you have a high pressure alarm? (PIP vs. Pplat)?
- If PIP is high but Pplat is not high, there might be problems with airway resistance e.g. kinked tube (for DDx refer to figure 30).
- You may need to suction down on the tube to figure out what is happening there.
- If Both PIP and Pplat are high: It signifies decreased lung compliance e.g. ARDS (for DDx refer to figure 30).
- If PIP is high but Pplat is not high, there might be problems with airway resistance e.g. kinked tube (for DDx refer to figure 30).
- If PIP is low. It can be caused by:
- Disconnection
- ETT cuff deflated
- Patient’s effort
- Do you have a low volume alarm going off?
- Endotracheal leak
- Bronchopleural Fistula
- Patient dyssynchrony? Is the patient fighting the ventilator?
- The ventilator waveform looks scalloping, just like what an ECG in a shivering patient looks like! Oftentimes sedation and paralysis resolve the problem.
- Breath stacking?
- Look at the ventilator flow-time waveform to figure out a breath stacking.
- In the absence of the above condition, if the ventilator is still alarming like “oxygen not connected” etc; you may have equipment problems and need to call a respiratory therapist!
- Possible problems: not hooked up, gases messed up, sensor messed up.
Ask the nurse: Obtain a focused history
- Indication for intubation?
- Difficulty of the intubation?
- Depth of the ET tube?
- Ventilator settings?
- Recent procedures or moves (central line insertion; chest tube placement; removal or transition to a water seal; thoracentesis; ET tube manipulation; transport off the stretcher; rotation for cleaning, a procedure, or chest radiography).
- Disconnected/reconnected circuit? (e.g. Do they recently put the patient on a transport ventilator and took the patient to CT?).
- If yes, derecruitment has happened upon disconnecting and reconnecting the patient.
- Have they recently moved the patient? (tube can be dislodged)
- Disconnected/reconnected circuit? (e.g. Do they recently put the patient on a transport ventilator and took the patient to CT?).
You may need to do the following
- Suction. If you cannot pass down the suction tube, there’s a problem down there like mucus plug, kinked tube etc.
- Ambu-bag the patient. It takes the equipment failure out of running, moreover it gives you a good feel for a patient’s lungs (how compliant and stiff their lungs are). Do you have airway resistance?
- Ultrasound: Provides helpful diagnostic clues to identify the following conditions:
- ETT displacement
- Pneumothorax
- Pulmonary edema
- Pleural effusion with or without atelectasis, or consolidation.
- Pulmonary embolism: Evidence of RV strain, proximal DVT
- Extrapulmonary causes of cardiorespiratory failure such as:
- Ascites
- Pericardial tamponade
- Pneumoperitoneum
- Ruptured aortic aneurysm
- ABG
RECAP
- Do not intubate a patient and leave the room! You should be able to set the vent at least initially.
- VC-AC and SIMV are the two most frequently used modes. Know how to work with these and master it.
- The basic ventilator parameters turn out to be limited to “tidal volume, RR, FiO2 and PEEP”. The first two primarily improve ventilation (PaCO2) and the latter two improve oxygenation (PaO2/FiO2).
- Remember that Peak inspiratory pressure (PIP) differs from plateau pressure(Pplat).
- Know how to figure out plateau pressure in your ventilator and appreciate its clinical significance.You should press the inspiratory hold button for 0.5 sec to figure out the Pplat.Check it after initial settings and at regular intervals thereafter (e.g. q4 hours or after each change in VT or PEEP).
- Do not be afraid of PEEP! In patients with lung injury, best individualized PEEP improves oxygenation via recruitment , and prevents ventilator-induced lung injury (atelectrauma, shear strain)
- Tidal volume is calculated per height (i.e.PBW). Remember that the patient’s lung size relates to his or her height but not the weight.
- Two primary ventilator management strategies involve lung injury (e.g. ARDS) and obstructive physiology (e.g. COPD).
- Ventilator management strategy for lung injury is based on applying low tidal volume. In fact, a protective lung strategy is good for every patient!…
- Keep in mind that ventilators show peak inspiratory pressure which is less relevant to the actual pressure that alveoli are experiencing. What most matters is the plateau pressure (Pplat). Commonly it is desired to keep Pplat ≤30 cm H2O.
- A high plateau pressure may signify non-compliant lung and/or worsening lung injury. Keep lowering the Vt until Plat <30. You may need to go as low as 4 cc/kg.
- The goal of strategy for obstructive physiology (COPD, asthma) is to give expiratory time as much as possible.
- Use a larger endotracheal tube (e.g. ≥8 mm) for adult patients with COPD/asthma.
- Remember that both extending the inspiratory time and increasing the RR; can contribute to breath stacking.
- Be tolerant to permissive hypercapnia (treat the patient but not the numbers). Do not sacrifice the lung for making the numbers look superficially pretty.
- Adjust the flow rate (in VC mode) and bring comfort to your patient!
- Ventilator alarm should be treated like a code blue announcement. This should be instilled into your culture. Figure out what’s going wrong and happening there!
- Do not forget to provide sedation and analgesics for your patients.
- Print this handout (By Scott Weingart).
Media
Effect of PEEP on lung expansion and alveolar recruitment
Appropriate method of prone positioning
Going further
- The Post-Intubation Package (EMCrit)
- Dominating the ventilator part 1 and part 2 (EMCrit)
- Ventilator Management Summary (EM:RAP)
- Vent Alarms = Code Blue (EMCrit)
- Coding Asthmatic, DOPES and Finger Thoracostomy(EMCrit)
- Alarms from the ventilator (ALIEM)
References
1.Silva PL, Rocco PRM. The basics of respiratory mechanics: ventilator-derived parameters. Ann Transl Med. 2018 Oct;6(19):376. doi: 10.21037/atm.2018.06.06. PMID: 30460250; PMCID: PMC6212352.
2. Williams EC, Motta-Ribeiro GC, Vidal Melo MF. Driving Pressure and Transpulmonary Pressure: How Do We Guide Safe Mechanical Ventilation? Anesthesiology. 2019 Jul;131(1):155-163. doi: 10.1097/ALN.0000000000002731. PMID: 31094753; PMCID: PMC6639048.
3. Gattinoni L, Busana M, Giosa L, Macrì MM, Quintel M. Prone Positioning in Acute Respiratory Distress Syndrome. Semin Respir Crit Care Med. 2019 Feb;40(1):94-100. doi: 10.1055/s-0039-1685180. Epub 2019 May 6. PMID: 31060091.
4. O’Driscoll BR, Howard LS, Earis J, Mak V; British Thoracic Society Emergency Oxygen Guideline Group; BTS Emergency Oxygen Guideline Development Group. BTS guideline for oxygen use in adults in healthcare and emergency settings. Thorax. 2017 Jun;72(Suppl 1):ii1-ii90. doi: 10.1136/thoraxjnl-2016-209729. PMID: 28507176.
5. Villar J, Martín-Rodríguez C, Domínguez-Berrot AM, Fernández L, Ferrando C, Soler JA, Díaz-Lamas AM, González-Higueras E, Nogales L, Ambrós A, Carriedo D, Hernández M, Martínez D, Blanco J, Belda J, Parrilla D, Suárez-Sipmann F, Tarancón C, Mora-Ordoñez JM, Blanch L, Pérez-Méndez L, Fernández RL, Kacmarek RM; Spanish Initiative for Epidemiology, Stratification and Therapies for ARDS (SIESTA) Investigators Network. A Quantile Analysis of Plateau and Driving Pressures: Effects on Mortality in Patients With Acute Respiratory Distress Syndrome Receiving Lung-Protective Ventilation. Crit Care Med. 2017 May;45(5):843-850. doi: 10.1097/CCM.0000000000002330. PMID: 28252536
6. Lei, Y. (2017). Medical Ventilator System Basics. In British Library Cataloguing. https://doi.org/10.1093/med/9780198784975.001.0001
7. Yang SC, Yang SP. Effects of inspiratory flow waveforms on lung mechanics, gas exchange, and respiratory metabolism in COPD patients during mechanical ventilation. Chest. 2002 Dec;122(6):2096-104. doi: 10.1378/chest.122.6.2096. PMID: 12475853
8. Sassoon CS, Del Rosario N, Fei R, Rheeman CH, Gruer SE, Mahutte CK. Influence of pressure- and flow-triggered synchronous intermittent mandatory ventilation on inspiratory muscle work. Crit Care Med. 1994 Dec;22(12):1933-41. PMID: 7988129
9. Aslanian P, El Atrous S, Isabey D, Valente E, Corsi D, Harf A, Lemaire F, Brochard L. Effects of flow triggering on breathing effort during partial ventilatory support. Am J Respir Crit Care Med. 1998 Jan;157(1):135-43. doi: 10.1164/ajrccm.157.1.96-12052. PMID: 9445291
10. Acute Respiratory Distress Syndrome Network, Brower RG, Matthay MA, Morris A, Schoenfeld D, Thompson BT, Wheeler A. Ventilation with lower tidal volumes as compared with traditional tidal volumes for acute lung injury and the acute respiratory distress syndrome. N Engl J Med. 2000 May 4;342(18):1301-8. doi: 10.1056/NEJM200005043421801. PMID: 10793162.
11. Beitler JR, Malhotra A, Thompson BT. Ventilator-induced Lung Injury. Clin Chest Med. 2016;37(4):633-646. doi:10.1016/j.ccm.2016.07.004
12. Caironi P, Cressoni M, Chiumello D, Ranieri M, Quintel M, Russo SG, Cornejo R, Bugedo G, Carlesso E, Russo R, Caspani L, Gattinoni L. Lung opening and closing during ventilation of acute respiratory distress syndrome. Am J Respir Crit Care Med. 2010 Mar 15;181(6):578-86. doi: 10.1164/rccm.200905-0787OC. Epub 2009 Nov 12. PMID: 19910610
13. Gattinoni L, Pesenti A. The concept of “baby lung”. Intensive Care Med. 2005 Jun;31(6):776-84. doi: 10.1007/s00134-005-2627-z. Epub 2005 Apr 6. PMID: 15812622
14. Boussarsar M, Thierry G, Jaber S, Roudot-Thoraval F, Lemaire F, Brochard L. Relationship between ventilatory settings and barotrauma in acute respiratory distress syndrome. Intensive Care Med. 2002 Apr;28(4):406-13. doi: 10.1007/s00134-001-1178-1. Epub 2002 Jan 15. PMID: 11967593
15. Brower RG, Lanken PN, MacIntyre N, Matthay MA, Morris A, Ancukiewicz M, Schoenfeld D, Thompson BT; National Heart, Lung, and Blood Institute ARDS Clinical Trials Network. Higher versus lower positive end-expiratory pressures in patients with the acute respiratory distress syndrome. N Engl J Med. 2004 Jul 22;351(4):327-36. doi: 10.1056/NEJMoa032193. PMID: 15269312
16. Gattinoni L, Protti A, Caironi P, Carlesso E. Ventilator-induced lung injury: the anatomical and physiological framework. Crit Care Med. 2010 Oct;38(10 Suppl):S539-48. doi: 10.1097/CCM.0b013e3181f1fcf7. PMID: 21164395
17. Sugiura M, McCulloch PR, Wren S, Dawson RH, Froese AB. Ventilator pattern influences neutrophil influx and activation in atelectasis-prone rabbit lung. J Appl Physiol (1985). 1994 Sep;77(3):1355-65. doi: 10.1152/jappl.1994.77.3.1355. PMID: 7836140.
18. Tremblay LN, Slutsky AS. Ventilator-induced lung injury: from the bench to the bedside. Intensive Care Med. 2006 Jan;32(1):24-33. doi: 10.1007/s00134-005-2817-8. Epub 2005 Oct 18. PMID: 16231069
19. Gattinoni L, Pelosi P, Crotti S, Valenza F. Effects of positive end-expiratory pressure on regional distribution of tidal volume and recruitment in adult respiratory distress syndrome. Am J Respir Crit Care Med. 1995 Jun;151(6):1807-14. doi: 10.1164/ajrccm.151.6.7767524. PMID: 7767524.
20. Weingart SD. Managing Initial Mechanical Ventilation in the Emergency Department. Ann Emerg Med. 2016 Nov;68(5):614-617. doi: 10.1016/j.annemergmed.2016.04.059. Epub 2016 Jun 9. PMID: 27289336
21. Guérin C, Reignier J, Richard JC, Beuret P, Gacoin A, Boulain T, Mercier E, Badet M, Mercat A, Baudin O, Clavel M, Chatellier D, Jaber S, Rosselli S, Mancebo J, Sirodot M, Hilbert G, Bengler C, Richecoeur J, Gainnier M, Bayle F, Bourdin G, Leray V, Girard R, Baboi L, Ayzac L; PROSEVA Study Group. Prone positioning in severe acute respiratory distress syndrome. N Engl J Med. 2013 Jun 6;368(23):2159-68. doi: 10.1056/NEJMoa1214103. Epub 2013 May 20. PMID: 23688302.
22. Nieman GF, Satalin J, Kollisch-Singule M, Andrews P, Aiash H, Habashi NM, Gatto LA. Physiology in Medicine: Understanding dynamic alveolar physiology to minimize ventilator-induced lung injury. J Appl Physiol (1985). 2017 Jun 1;122(6):1516-1522. doi: 10.1152/japplphysiol.00123.2017. Epub 2017 Apr 6. PMID: 28385915; PMCID: PMC7203565
23. Slutsky AS, Ranieri VM. Ventilator-induced lung injury. N Engl J Med. 2013 Nov 28;369(22):2126-36. doi: 10.1056/NEJMra1208707. Erratum in: N Engl J Med. 2014 Apr 24;370(17):1668-9. PMID: 24283226
24. Hughes KT, Beasley MB. Pulmonary Manifestations of Acute Lung Injury: More Than Just Diffuse Alveolar Damage. Arch Pathol Lab Med. 2017 Jul;141(7):916-922. doi: 10.5858/arpa.2016-0342-RA. Epub 2016 Sep 21. PMID: 27652982
25. Cvach MM, Stokes JE, Manzoor SH, Brooks PO, Burger TS, Gottschalk A, Pustovoit A. Ventilator Alarms in Intensive Care Units: Frequency, Duration, Priority, and Relationship to Ventilator Parameters. Anesth Analg. 2020 Jan;130(1):e9-e13. doi: 10.1213/ANE.0000000000003801. PMID: 30234538.
26. Guérin C, Reignier J, Richard JC, Beuret P, Gacoin A, Boulain T, Mercier E, Badet M, Mercat A, Baudin O, Clavel M, Chatellier D, Jaber S, Rosselli S, Mancebo J, Sirodot M, Hilbert G, Bengler C, Richecoeur J, Gainnier M, Bayle F, Bourdin G, Leray V, Girard R, Baboi L, Ayzac L; PROSEVA Study Group. Prone positioning in severe acute respiratory distress syndrome. N Engl J Med. 2013 Jun 6;368(23):2159-68. doi: 10.1056/NEJMoa1214103. Epub 2013 May 20. PMID: 23688302
27. Fan E, Del Sorbo L, Goligher EC, Hodgson CL, Munshi L, Walkey AJ, Adhikari NKJ, Amato MBP, Branson R, Brower RG, Ferguson ND, Gajic O, Gattinoni L, Hess D, Mancebo J, Meade MO, McAuley DF, Pesenti A, Ranieri VM, Rubenfeld GD, Rubin E, Seckel M, Slutsky AS, Talmor D, Thompson BT, Wunsch H, Uleryk E, Brozek J, Brochard LJ; American Thoracic Society, European Society of Intensive Care Medicine, and Society of Critical Care Medicine. An Official American Thoracic Society/European Society of Intensive Care Medicine/Society of Critical Care Medicine Clinical Practice Guideline: Mechanical Ventilation in Adult Patients with Acute Respiratory Distress Syndrome. Am J Respir Crit Care Med. 2017 May 1;195(9):1253-1263. doi: 10.1164/rccm.201703-0548ST. Erratum in: Am J Respir Crit Care Med. 2017 Jun 1;195(11):1540. PMID: 28459336.
28. Meade MO, Cook DJ, Guyatt GH, Slutsky AS, Arabi YM, Cooper DJ, Davies AR, Hand LE, Zhou Q, Thabane L, Austin P, Lapinsky S, Baxter A, Russell J, Skrobik Y, Ronco JJ, Stewart TE; Lung Open Ventilation Study Investigators. Ventilation strategy using low tidal volumes, recruitment maneuvers, and high positive end-expiratory pressure for acute lung injury and acute respiratory distress syndrome: a randomized controlled trial. JAMA. 2008 Feb 13;299(6):637-45. doi: 10.1001/jama.299.6.637. PMID: 18270352
29. Fan E, Brodie D, Slutsky AS. Acute Respiratory Distress Syndrome: Advances in Diagnosis and Treatment. JAMA. 2018 Feb 20;319(7):698-710. doi: 10.1001/jama.2017.21907. PMID: 29466596
30. Kregenow DA, Rubenfeld GD, Hudson LD, Swenson ER. Hypercapnic acidosis and mortality in acute lung injury. Crit Care Med. 2006 Jan;34(1):1-7. doi: 10.1097/01.ccm.0000194533.75481.03. PMID: 16374149
31. Amato MB, Meade MO, Slutsky AS, Brochard L, Costa EL, Schoenfeld DA, Stewart TE, Briel M, Talmor D, Mercat A, Richard JC, Carvalho CR, Brower RG. Driving pressure and survival in the acute respiratory distress syndrome. N Engl J Med. 2015 Feb 19;372(8):747-55. doi: 10.1056/NEJMsa1410639. PMID: 25693014
32. Tonetti T, Vazquez F, Rapetti F, Maiolo G, Collino F, Romitti F, Camporota L, Cressoni M, Cadringher P, Quintel M, Gattinoni L. Driving pressure and mechanical power: new targets for VILI prevention. Ann Transl Med. 2017 July;5(14):286. doi: 10.21037/atm.2017.07.08. PMID: 28828361; PMCID: PMC5537108
33. Amato MB, Meade MO, Slutsky AS, Brochard L, Costa EL, Schoenfeld DA, Stewart TE, Briel M, Talmor D, Mercat A, Richard JC, Carvalho CR, Brower RG. Driving pressure and survival in the acute respiratory distress syndrome. N Engl J Med. 2015 Feb 19;372(8):747-55. doi: 10.1056/NEJMsa1410639. PMID: 25693014.
34. Hubmayr RD, Pannu S. Understanding lung protection. Intensive Care Med. 2015 Dec;41(12):2184-6. doi: 10.1007/s00134-015-4100-y. PMID: 26499478.
35. Villar J, Martín-Rodríguez C, Domínguez-Berrot AM, Fernández L, Ferrando C, Soler JA, Díaz-Lamas AM, González-Higueras E, Nogales L, Ambrós A, Carriedo D, Hernández M, Martínez D, Blanco J, Belda J, Parrilla D, Suárez-Sipmann F, Tarancón C, Mora-Ordoñez JM, Blanch L, Pérez-Méndez L, Fernández RL, Kacmarek RM; Spanish Initiative for Epidemiology, Stratification and Therapies for ARDS (SIESTA) Investigators Network. A Quantile Analysis of Plateau and Driving Pressures: Effects on Mortality in Patients With Acute Respiratory Distress Syndrome Receiving Lung-Protective Ventilation. Crit Care Med. 2017 May;45(5):843-850. doi: 10.1097/CCM.0000000000002330. PMID: 28252536.
36. Pelosi P, Ball L. Should we titrate ventilation based on driving pressure? Maybe not in the way we would expect. Ann Transl Med. 2018 Oct;6(19):389. doi: 10.21037/atm.2018.09.48. PMID: 30460263; PMCID: PMC6212354.
37. Talmor D, Sarge T, Malhotra A, O’Donnell CR, Ritz R, Lisbon A, Novack V, Loring SH. Mechanical ventilation guided by esophageal pressure in acute lung injury. N Engl J Med. 2008 Nov 13;359(20):2095-104. doi: 10.1056/NEJMoa0708638. Epub 2008 Nov 11. PMID: 19001507; PMCID: PMC3969885
38. Ahmed SM, Athar M. Mechanical ventilation in patients with chronic obstructive pulmonary disease and bronchial asthma. Indian J Anaesth. 2015 Sep;59(9):589-98. doi: 10.4103/0019-5049.165856. PMID: 26556918; PMCID: PMC4613406.
39. Feihl F, Perret C. Permissive hypercapnia. How permissive should we be? Am J Respir Crit Care Med. 1994 Dec;150(6 Pt 1):1722-37. doi: 10.1164/ajrccm.150.6.7952641. PMID: 7952641.
40. Bidani A, Tzouanakis AE, Cardenas VJ Jr, Zwischenberger JB. Permissive hypercapnia in acute respiratory failure. JAMA. 1994 Sep 28;272(12):957-62. PMID: 8084064.
41. Lei, Y. (2017). Alarms and Safety Mechanisms Medical Ventilator System Basics : A. https://doi.org/10.1093/med/9780198784975.001.0001
42. Cvach MM, Stokes JE, Manzoor SH, Brooks PO, Burger TS, Gottschalk A, Pustovoit A. Ventilator Alarms in Intensive Care Units: Frequency, Duration, Priority, and Relationship to Ventilator Parameters. Anesth Analg. 2020 Jan;130(1):e9-e13. doi: 10.1213/ANE.0000000000003801. PMID: 30234538.
43. De Jong A, Verzilli D, Jaber S. ARDS in Obese Patients: Specificities and Management. Crit Care. 2019;23(1):74. Published 2019 Mar 9. doi:10.1186/s13054-019-2374-0
44. Wilcox, S. R., Aydin, A., Marcolini, E. G., Wilcox, S. R., Aydin, A., & Marcolini, E. G. (2019). Chapter 9.Specific Circumstances:Asthma and COPD; Correction to: Mechanical Ventilation in Emergency Medicine. In Mechanical Ventilation in Emergency Medicine. https://doi.org/10.1007/978-3-319-98410-0_14
Add comment